New Material Could Improve Gastrointestinal Drug Delivery of Medicines
The oral route is by far the most common route for drug administration in the gastrointestinal tract (GI tract) and can be used for both systemic drug delivery and for treating local gastrointestinal diseases. It is the most preferred route by patients, due to its advantages, such as ease of use, non-invasiveness, and convenience for self-administration. Drugs administered via the oral route, however, generally have slower absorption, which is not preferred during an emergency. They might also be unpleasant in taste, cause gastric irritation, and/or undergo first-pass drug elimination processes in both the intestine and liver. In addition, the physiological environment in the GI tract can also affect the stability and solubility of drugs.
Considering the large pH gradient between the stomach and the intestines, platform technologies that control the retention and release of material using this large gradient can improve the delivery of cargo to areas distal to the stomach. This problem is of great medical interest because currently, many oral drug delivery technologies suffer from significant degradation upon exposure to the harsh gastric environment. As a result, many modern therapeutics cannot be formulated as orally-delivered drugs and can instead only be delivered intravenously. Avoiding gastric degradation is especially tantalizing because the small intestine is the site of greatest and most efficient absorption in the GI tract. Furthermore, intestinal conditions are much gentler, since the production of basic secretory fluids neutralizes its luminal pH. Hence, there is an opportunity for drug delivery technologies to take advantage of this significant pH differential by using it as a chemical trigger to release encapsulated cargo.
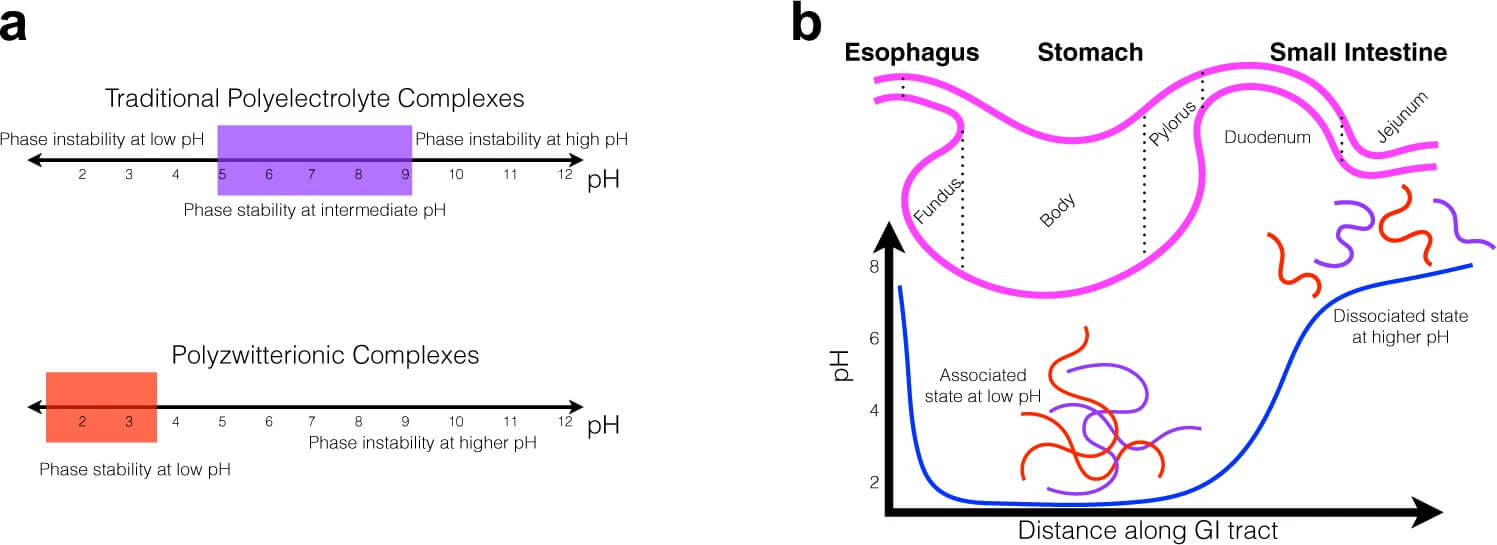
Researchers at the University of Massachusetts Amherst recently announced that they have engineered a new class of material, called a “polyzwitterionic complex,” or “pZC,” which is able to both withstand the harsh acidic conditions of the stomach and then dissolve predictably in the comparatively gentle environment of the small intestine. This property means that pZCs could help revolutionize the delivery of medicines of all sorts, from familiar oral antibiotics to new classes of delicate protein therapeutics.
Their idea is based on the theory of complexation between charged macromolecules. The two major contributions to inter-molecular complexation among polar and charged macromolecules are electrostatic interactions among all polar and charged repeat units of the complexing chains and entropy gain due to the release of counterions during complexation. To be specific, let the host molecule be poly(acrylic acid) (pKa ≃ 6.2) and the guest be the polyzwitterion poly(2-methacryloyloxyethyl phosphorylcholine) (pMPC) (pKa ≃ 2.3 for the phosphoryl group). Under the experimental conditions of interest here, namely pH < 4, polyacrylic acid can be treated as a dipolar chain. For pH < 4, the polyzwitterion is essentially a polycation, and for pH > 4, it is a dipolar chain.
Since the dipole-charge interaction, accompanied by counterion release, results in complexation and mere dipole-dipole interactions are not strong enough to lead to complexation, the researchers envisage a mechanism of devising a change from a complexed state to an uncomplexed state in the above mentioned pH range, by making the zwitterionic repeat unit of the guest charged at lower pH and dipolar at higher pH. Using the above guidance from nature, the team can justify their earlier choice of pMPC and pAA to explore the feasibility of this new class of materials, comprised of interacting chains of zwitterionic and anionic polymers, with respect to their specific applicability to the retention and release of cargo inside the gastrointestinal tract.
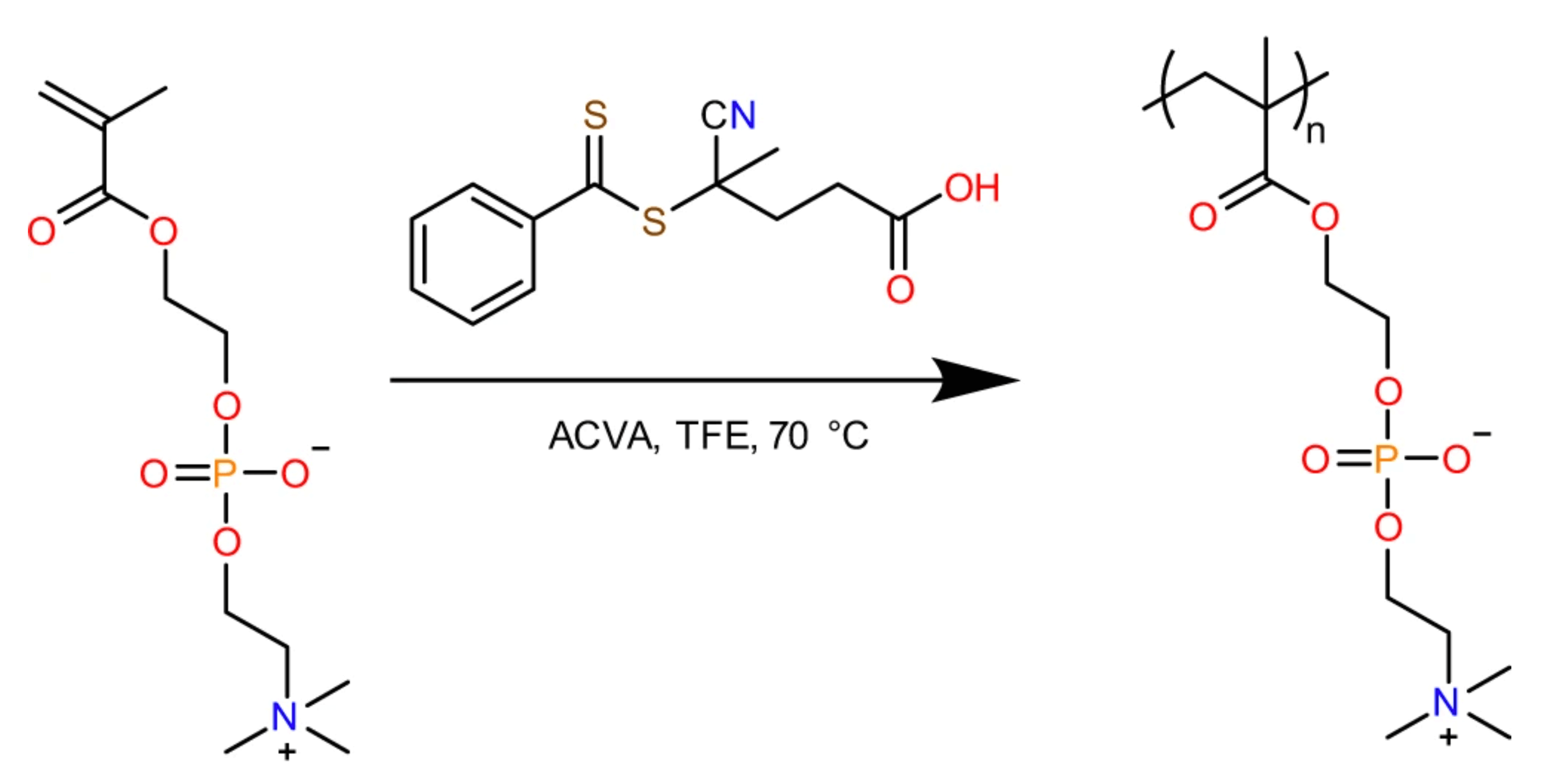
These complexes of pMPC and pAA demonstrate many of the same properties as those seen in polyelectrolyte coacervates, even though one of the components is a polyzwitterion, rather than a traditional polyelectrolyte. The researchers harnessed these properties to demonstrate the potential applicability of polyzwitterionic coacervates as platform technologies for the pH-triggered release of cargo. This work inspires numerous follow-up questions of both a fundamental and applied nature. Fundamentally, aspects of the phase behavior presented here have implications in complexation within other polyzwitterionic materials. Designing analogous synthetic polyzwitterions to tailor specific pH-responsive criteria could be one avenue of fruitful research. The chemistry can be adjusted to suit stability or instability requirements at particular pH values. Furthermore, switching the two charged groups on the zwitterionic monomer, such that the permanently charged functional group is negative, and the variably charged functional group is positive, should lead to inverse phase behavior relative to that described in this work, if such a polyzwitterion were paired with a traditional polycation.
The study, recently published in Nature Communications, details a new class of material, called a pZC, which forms through a process known as “complex coacervation”. In their system, two types of charged polymers, a polyzwitterion and a polyelectrolyte, associate to form a protective droplet inside of which medications can travel. The trick that the pZC has to perform is that it not only needs to be tough enough to withstand the highly acidic stomach environment, it also has to disassemble in the much gentler, neutral conditions of the small intestine.
List of drugs by gastrointestinal delivery (based on WHO Model Lists of Essential Medicines)
- Dapsone
- Calcium
- Tramadol
- Cefixime
- Nystatin
- Pyrantel
- Succimer
- Imatinib
- Losartan
- Abacavir
- Warfarin
- Thiamine
- Atenolol
- Linezolid
- Ibuprofen
- Phenytoin
- Stavudine
- Diazoxide
- Isoniazid
- Tamoxifen
- Proguanil
- Erlotinib
- Ibrutinib
- Rifabutin
- Nilotinib
- Bupropion
- Enalapril
- Dasatinib
- Ofloxacin
- Captopril
- Ritonavir
- Clozapine
- Dasabuvir
- Verapamil
- Indinavir
- Amiloride
- Clomifene
- Cyclizine
- Delamanid
- Metformin
- Efavirenz
- Darunavir
- Loratadine
- Didanosine
- Aprepitant
- Rifampicin
- Omeprazole
- Ethambutol
- Lamivudine
- Nevirapine
- Ranitidine
- Ropinirole
- Fingolimod
- Colchicine
- Trametinib
- Folic acid
- Bisoprolol
- Nifedipine
- Nifedipine
- Atazanavir
- Dabigatran
- Nifurtimox
- Vancomycin
- Simeprevir
- Loperamide
- Everolimus
- Fluoxetine
- Cimetidine
- Crizotinib
- Saquinavir
- Pyridoxine
- Pyridoxine
- Methyldopa
- Nelfinavir
- Mefloquine
- Ivermectin
- Gliclazide
- Ulipristal
- Sofosbuvir
- Amlodipine
- Levamisole
- Dabrafenib
- Probenecid
- Diloxanide
- Primaquine
- Raltegravir
- Cloxacillin
- Amoxicillin
- Paromomycin
- Paromomycin
- Haloperidol
- Doxycycline
- Oxamniquine
- Chloroquine
- Clindamycin
- Risperidone
- Cabergoline
- Varenicline
- Anastrozole
- Miltefosine
- Allopurinol
- Mebendazole
- Bedaquiline
- Clofazimine
- Ethionamide
- Pramipexole
- Neostigmine
- Rifapentine
- Amodiaquine
- Simvastatin
- Pentamidine
- Misoprostol
- Lamotrigine
- Cycloserine
- Abiraterone
- Oseltamivir
- Daclatasvir
- Osimertinib
- Propranolol
- Albendazole
- Methimazole
- Sumatriptan
- Thalidomide
- Tolbutamide
- Niclosamide
- Palbociclib
- Clopidogrel
- Itraconazole
- Griseofulvin
- Azithromycin
- Ethosuximide
- Pyrazinamide
- Promethazine
- Trimethoprim
- Clomipramine
- Benznidazole
- Capecitabine
- Chlorambucil
- Methotrexate
- Gatifloxacin
- Lenalidomide
- Tetracycline
- Levofloxacin
- Moxifloxacin
- Azathioprine
- Bicalutamide
- Nicotinamide
- Procarbazine
- Dolutegravir
- Sulfadiazine
- Fexinidazole
- Praziquantel
- Praziquantel
- Zanubrutinib
- Dexamethasone
- Emtricitabine
- Carbamazepine
- Metronidazole
- Empagliflozin
- Bromocriptine
- Pyrimethamine
- Amitriptyline
- Acetazolamide
- Ascorbic acid
- Levothyroxine
- Sulfasalazine
- Chlorpromazine
- Metoclopramide
- Valganciclovir
- Barium sulfate
- Nalidixic acid
- Mercaptopurine
- Clarithromycin
- Levonorgestrel
- Docusate sodium
- Fludrocortisone
- Trihexyphenidyl
- Triclabendazole
- Calcium folinate
- Cyclophosphamide
- Propylthiouracil
- Potassium iodide
- Potassium chloride
- Hydroxychloroquine
- Diethylcarbamazine
- Hydrochlorothiazide
References
- Hua, Susan. "Advances in oral drug delivery for regional targeting in the gastrointestinal tract-influence of physiological, pathophysiological and pharmaceutical factors." Frontiers in pharmacology 11 (2020): 524.
- Margossian, Khatcher O., Marcel U. Brown, Todd Emrick, and Murugappan Muthukumar. "Coarvation in polyzwitterion-polyelectrolyte systems and their potential applications for gastrointestinal drug delivery platforms." Nature Communications 13, no. 1 (2022): 1-11.