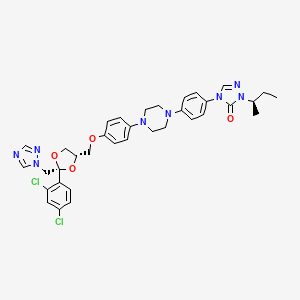
Itraconazole
Overview
Description
Itraconazole is a triazole antifungal agent used to treat a variety of fungal infections, including aspergillosis, blastomycosis, coccidioidomycosis, histoplasmosis, and paracoccidioidomycosis . It was first synthesized in the early 1980s and approved for medical use in the United States in 1992 . This compound is known for its broad-spectrum activity against fungal pathogens and is available in oral and intravenous formulations .
Mechanism of Action
Target of Action
Itraconazole primarily targets the fungal cytochrome P450 enzyme, lanosterol 14α-demethylase . This enzyme plays a crucial role in the conversion of lanosterol to ergosterol, a vital component of fungal cell membranes .
Mode of Action
This compound exerts its antifungal activity by inhibiting the synthesis of ergosterol . It forms a complex with the active site, or the haem iron, of the fungal enzyme lanosterol 14α-demethylase . This inhibition blocks the conversion of lanosterol to ergosterol, disrupting the integrity of the fungal cell membrane, leading to leakage of essential cellular components, and ultimately inhibiting fungal growth and replication .
Biochemical Pathways
The inhibition of ergosterol synthesis leads to an accumulation of 14-methylsterol and depletion of ergosterol in fungal cells . This results in disruptions in membrane structure and function, affecting the stability and activity of many membrane-bound enzymes, and preventing fungal growth .
Pharmacokinetics
This compound is extensively metabolized in the liver, primarily by the CYP3A4 enzyme . The major metabolite, hydroxy-itraconazole, reaches higher plasma concentrations than the parent compound and also has considerable antifungal activity . The bioavailability of this compound is approximately 55%, and it is maximal if taken with a full meal . The elimination half-life of this compound is about 21 hours , and it is excreted via the kidneys (35%) and feces (54%) .
Result of Action
The result of this compound’s action is the effective treatment of various fungal infections, including blastomycosis, histoplasmosis, and aspergillosis, in both immunocompromised and non-immunocompromised patients . It is also effective against onychomycosis of the toenail and fingernail due to dermatophytes, and for the treatment of oropharyngeal and esophageal candidiasis .
Action Environment
The action of this compound can be influenced by various environmental factors. For instance, the bioavailability of this compound is significantly increased when taken with food . Additionally, the drug’s efficacy can be affected by the patient’s immune status, with immunocompromised patients potentially requiring higher doses or longer treatment durations . Furthermore, the presence of other medications can influence this compound’s action due to potential drug-drug interactions, as this compound is known to inhibit the CYP3A4 enzyme, which is involved in the metabolism of many drugs .
Biochemical Analysis
Biochemical Properties
Itraconazole mediates its antifungal activity by inhibiting 14α-demethylase, a fungal cytochrome P450 enzyme that converts lanosterol to ergosterol . Ergosterol is a vital component of fungal cell membranes. The azole nitrogen atoms in the chemical structure of this compound form a complex with the active site, or the haem iron, of the fungal enzyme to impede its function .
Cellular Effects
This compound has been shown to inhibit the proliferation of certain cancer cells . It can regulate the G1-S transition and induce apoptosis of gastric cancer cells . In esophageal squamous cell carcinoma and adenocarcinoma cell lines, this compound inhibited cell proliferation and induced G1-phase cell-cycle arrest .
Molecular Mechanism
The mechanism of action of this compound is the same as the other azole antifungals: it inhibits the fungal-mediated synthesis of ergosterol, via inhibition of lanosterol 14α-demethylase . This results in the accumulation of lanosterol and 14-methylated sterols, leading to increased permeability of the fungal cell membrane, modified membrane-bound enzyme activity, and dysregulated chitin synthesis .
Temporal Effects in Laboratory Settings
In laboratory studies, this compound has shown a progressive increase in the probability of toxicity with increasing concentrations . The drug-induced changes of oxidative and peroxidative enzyme activities lead to toxic concentrations of hydrogen peroxide developing intracellularly .
Dosage Effects in Animal Models
In animal models, malformations and foetal resorptions were seen in overdose studies . High dosages (40 and 160 mg/kg bw/day for 10 days during their gestational period) have shown evidence of dose-related teratogenic, foetotoxic and maternotoxic effects .
Metabolic Pathways
This compound is extensively metabolized by the liver, predominantly by the CYP3A4 isoenzyme system . It undergoes extensive hepatic metabolism into more than 30 metabolites, including hydroxy-itraconazole, which appears to have in vitro antifungal activity .
Transport and Distribution
This compound is lipophilic and extensively distributed into tissues . Concentrations in the lung, kidney, liver, bone, stomach, spleen, and muscle were found to be two to three times higher than corresponding concentrations in plasma .
Subcellular Localization
It is known that this compound alters O-glycosylation and subcellular localization of CD44, a cell surface glycoprotein involved in cell-cell interactions, cell adhesion, and migration .
Preparation Methods
Synthetic Routes and Reaction Conditions: The synthesis of itraconazole involves multiple steps, starting with the preparation of key intermediates. One common method includes the reaction of 1-(2,4-dichlorophenyl)-2-(1H-1,2,4-triazol-1-yl)ethanone with 1,3-dioxolane to form a dioxolane intermediate. This intermediate is then reacted with piperazine to yield this compound .
Industrial Production Methods: In industrial settings, this compound is prepared by dissolving a mixture of this compound and L-ascorbic acid in a mixed solvent of dichloromethane and methanol. Hydroxypropyl methylcellulose and pluronic F-127 are added, followed by dichloromethane to obtain a raw material solution. This solution is then subjected to supercritical fluid crystallization using carbon dioxide, resulting in composite particles with decreased particle size and increased bioavailability .
Chemical Reactions Analysis
Types of Reactions: Itraconazole undergoes several types of chemical reactions, including oxidation, reduction, and substitution.
Common Reagents and Conditions:
Reduction: Specific reduction reactions of this compound are less common in the literature.
Substitution: Substitution reactions involving this compound typically occur during its synthesis rather than in its metabolic pathways.
Major Products Formed: The major metabolites of this compound include hydroxy-itraconazole, keto-itraconazole, and N-desalkyl-itraconazole .
Scientific Research Applications
Itraconazole has a wide range of scientific research applications:
Chemistry: this compound is studied for its unique chemical properties and interactions with various reagents.
Biology: It is used to investigate fungal cell biology and the mechanisms of antifungal resistance.
Medicine: this compound is extensively used in clinical settings to treat fungal infections.
Industry: this compound is used in the formulation of antifungal medications and topical treatments.
Comparison with Similar Compounds
Itraconazole is often compared with other triazole antifungal agents such as fluconazole, voriconazole, and posaconazole:
Fluconazole: this compound has a broader spectrum of activity, particularly against Aspergillus species, which fluconazole does not cover.
Voriconazole: Voriconazole has a broader spectrum of activity than this compound but is associated with more severe side effects.
Posaconazole: Posaconazole is similar to this compound in terms of spectrum but has better bioavailability and fewer drug interactions.
This compound’s unique properties, such as its broad-spectrum activity and potential use in cancer treatment, make it a valuable compound in both clinical and research settings.
Properties
CAS No. |
84625-61-6 |
---|---|
Molecular Formula |
C35H38Cl2N8O4 |
Molecular Weight |
705.6 g/mol |
IUPAC Name |
2-butan-2-yl-4-[4-[4-[4-[[(2R)-2-(2,4-dichlorophenyl)-2-(1,2,4-triazol-1-ylmethyl)-1,3-dioxolan-4-yl]methoxy]phenyl]piperazin-1-yl]phenyl]-1,2,4-triazol-3-one |
InChI |
InChI=1S/C35H38Cl2N8O4/c1-3-25(2)45-34(46)44(24-40-45)29-7-5-27(6-8-29)41-14-16-42(17-15-41)28-9-11-30(12-10-28)47-19-31-20-48-35(49-31,21-43-23-38-22-39-43)32-13-4-26(36)18-33(32)37/h4-13,18,22-25,31H,3,14-17,19-21H2,1-2H3/t25?,31?,35-/m0/s1 |
InChI Key |
VHVPQPYKVGDNFY-WSTHBRJPSA-N |
Isomeric SMILES |
CCC(C)N1C(=O)N(C=N1)C2=CC=C(C=C2)N3CCN(CC3)C4=CC=C(C=C4)OCC5CO[C@](O5)(CN6C=NC=N6)C7=C(C=C(C=C7)Cl)Cl |
impurities |
Impurities: 4-[4-[4-(4-methoxyphenyl)piperazin-1-yl]phenyl]-2-[(1RS)-1-methylpropyl]-2,4-dihydro-3H-1,2,4-triazol-3-one; 4-[4-[4-[4-[[cis-2-(2,4-dichlorophenyl)-2-(4H-1,2,4-triazol-4-ylmethyl)-1,3-dioxolan-4-yl]methoxy]phenyl]piperazin-1-yl]phenyl]-2-[(1RS)-1-methylpropyl]-2,4-dihydro-3H-1,2,4-triazol-3-one; 4-[4-[4-[4-[[cis-2-(2,4-dichlorophenyl)-2-(1H-1,2,4-triazol-1-ylmethyl)-1,3-dioxolan-4-yl]methoxy]phenyl]piperazin-1-yl]phenyl]-2-propyl-2,4-dihydro-3H-1,2,4-triazol-3-one; 4-[4-[4-[4-[[cis-2-(2,4-dichlorophenyl)-2-(1H-1,2,4-triazol-1-ylmethyl)-1,3-dioxolan-4-yl]methoxy]phenyl]piperazin-1-yl]phenyl]-2-(1-methylethyl)-2,4-dihydro-3H-1,2,4-triazol-3-one; 4-[4-[4-[4-[[trans-2-(2,4-dichlorophenyl)-2-(1H-1,2,4-triazol-1-ylmethyl)-1,3-dioxolan-4-yl]methoxy]phenyl]piperazin-1-yl]phenyl]-2-[(1RS)-1-methylpropyl]-2,4-dihydro-3H-1,2,4-triazol-3-one; 2-butyl-4-[4-[4-[4-[[cis-2-(2,4-dichlorophenyl)-2-(1H-1,2,4-triazol-1-ylmethyl)-1,3-dioxolan-4-yl]methoxy]phenyl]piperazin-1-yl]phenyl]-2,4-dihydro-3H-1,2,4-triazol-3-one; 4-[4-[4-[4-[[cis-2-(2,4-dichlorophenyl)-2-(1H-1,2,4-triazol-1-ylmethyl)-1,3-dioxolan-4-yl]methoxy]phenyl]piperazin-1-yl]phenyl]-2-[[cis-2-(2,4-dichlorophenyl)-2-(1H-1,2,4-triazol-1-ylmethyl)-1,3-dioxolan-4-yl]methyl]-2,4-dihydro-3H-1,2,4-triazol-3-one. |
SMILES |
CCC(C)N1C(=O)N(C=N1)C2=CC=C(C=C2)N3CCN(CC3)C4=CC=C(C=C4)OCC5COC(O5)(CN6C=NC=N6)C7=C(C=C(C=C7)Cl)Cl |
Canonical SMILES |
CCC(C)N1C(=O)N(C=N1)C2=CC=C(C=C2)N3CCN(CC3)C4=CC=C(C=C4)OCC5COC(O5)(CN6C=NC=N6)C7=C(C=C(C=C7)Cl)Cl |
Appearance |
Solid powder |
Color/Form |
Solid Crystals from toluene |
melting_point |
168-170 166.2 °C |
84625-61-6 84604-65-9 |
|
physical_description |
Solid |
Pictograms |
Irritant |
Purity |
>98% (or refer to the Certificate of Analysis) |
shelf_life |
>2 years if stored properly |
solubility |
Practically insoluble in water and dilute acidic solutions |
storage |
Dry, dark and at 0 - 4 C for short term (days to weeks) or -20 C for long term (months to years). |
Synonyms |
Itraconazole, R51211, Orungal, Oriconazole, Sporanox, Itraconazolum, Itraconazol, Itrizole |
vapor_pressure |
2.6X10-20 mm Hg at 25 °C (est) |
Origin of Product |
United States |
Retrosynthesis Analysis
AI-Powered Synthesis Planning: Our tool employs the Template_relevance Pistachio, Template_relevance Bkms_metabolic, Template_relevance Pistachio_ringbreaker, Template_relevance Reaxys, Template_relevance Reaxys_biocatalysis model, leveraging a vast database of chemical reactions to predict feasible synthetic routes.
One-Step Synthesis Focus: Specifically designed for one-step synthesis, it provides concise and direct routes for your target compounds, streamlining the synthesis process.
Accurate Predictions: Utilizing the extensive PISTACHIO, BKMS_METABOLIC, PISTACHIO_RINGBREAKER, REAXYS, REAXYS_BIOCATALYSIS database, our tool offers high-accuracy predictions, reflecting the latest in chemical research and data.
Strategy Settings
Precursor scoring | Relevance Heuristic |
---|---|
Min. plausibility | 0.01 |
Model | Template_relevance |
Template Set | Pistachio/Bkms_metabolic/Pistachio_ringbreaker/Reaxys/Reaxys_biocatalysis |
Top-N result to add to graph | 6 |
Feasible Synthetic Routes
Q1: What is the primary mechanism of action of Itraconazole?
A1: this compound exerts its antifungal activity by inhibiting the fungal cytochrome P450-dependent enzyme lanosterol 14α-demethylase. [] This enzyme plays a crucial role in the biosynthesis of ergosterol, a vital component of the fungal cell membrane. []
Q2: What are the downstream effects of inhibiting lanosterol 14α-demethylase?
A2: Inhibiting lanosterol 14α-demethylase disrupts ergosterol synthesis, leading to the accumulation of 14α-methylated sterol precursors within the fungal cell. [] This accumulation has several detrimental effects on the fungus:
- Membrane Integrity Disruption: The altered sterol composition compromises the integrity and fluidity of the fungal cell membrane. []
- Growth Inhibition: The inability to synthesize ergosterol ultimately inhibits fungal cell growth and replication. [, ]
Q3: What is the molecular formula and weight of this compound?
A3: this compound possesses the molecular formula C35H38Cl2N8O4 and a molecular weight of 705.64 g/mol.
Q4: Are there any specific spectroscopic data available for this compound?
A4: While the provided research papers do not delve into detailed spectroscopic data, high-performance liquid chromatography (HPLC) coupled with various detection techniques like UV [] and fluorescence [] are commonly employed for this compound analysis. Mass spectrometry (MS), particularly in tandem with HPLC (LC-MS/MS), is also frequently used for sensitive quantification. []
Q5: How does the formulation of this compound affect its stability and bioavailability?
A5: this compound exhibits variable bioavailability due to its low aqueous solubility. [] Studies have shown that administering this compound with food can enhance its absorption and bioavailability. [] Developing formulations like nanoparticles [] and transethosomes [] can improve solubility and enhance delivery to target tissues.
Q6: How is this compound metabolized in the body?
A6: this compound undergoes extensive metabolism primarily in the liver by the cytochrome P450 enzyme, specifically CYP3A4. [, ] One major active metabolite is hydroxythis compound, often found in plasma at higher concentrations than the parent drug. [, ]
Q7: How does this compound interact with other drugs that are also metabolized by CYP3A4?
A7: As a potent CYP3A4 inhibitor, this compound can significantly affect the metabolism of co-administered drugs that rely on this enzyme. [, , ] This inhibition can lead to increased plasma concentrations of these drugs, potentially causing adverse effects or toxicity. [, , ] Some notable interactions include:
- Corticosteroids: this compound can increase the levels of inhaled and oral corticosteroids, potentially leading to Cushing's syndrome or adrenal insufficiency. []
- Tacrolimus: Co-administration of this compound can elevate tacrolimus concentrations, increasing the risk of nephrotoxicity. []
- Fexofenadine: this compound can significantly increase the exposure to fexofenadine, even at lower doses. []
Q8: What is the significance of hydroxythis compound in the overall antifungal activity of this compound?
A8: Hydroxythis compound, the major active metabolite, exhibits comparable antifungal activity to this compound in vitro. [, ] Its presence in plasma at higher concentrations than the parent drug contributes significantly to the overall efficacy of this compound treatment. []
Q9: How does this compound compare with other antifungal agents in terms of efficacy against various fungal species?
A9: this compound demonstrates a broad spectrum of activity against various fungal species:
- Aspergillus species: Effective against many Aspergillus species, but resistance has been observed. [, ] Combining this compound with agents like domiphen may combat this resistance. []
- Sporothrix schenckii: Demonstrates efficacy in treating sporotrichosis, although relapse can occur. []
- Cryptococcus neoformans: Effective in inhibiting ergosterol synthesis and exhibits good activity against this species. []
- Dimorphic Fungi: Effective against both yeast and mycelial forms of dimorphic fungi. []
- Zygomycetes & Fusarium species: Generally less active against these fungal groups. [, ]
Q10: What in vivo models are used to study the efficacy of this compound?
A10: Murine models are commonly employed to evaluate this compound efficacy against systemic fungal infections like candidiasis and histoplasmosis. [, ] These models provide valuable insights into the drug's ability to reduce fungal burden and improve survival rates.
Q11: What are the mechanisms of resistance to this compound?
A11: Resistance to this compound can arise through several mechanisms:
- Efflux pump overexpression: Upregulation of drug efflux pumps can actively remove this compound from the fungal cell, reducing its intracellular concentration. []
- Biofilm formation: Fungi within biofilms exhibit reduced susceptibility to antifungal agents, including this compound. []
Q12: What are some strategies employed to enhance the delivery of this compound to target tissues?
A12: Due to its low aqueous solubility, researchers are exploring different drug delivery strategies:
- Nanoparticles: Encapsulating this compound within nanoparticles can improve its solubility and bioavailability, allowing for targeted delivery to specific tissues. []
- Transethosomes: These specialized vesicles can enhance transdermal delivery of this compound, enabling localized treatment of skin infections. []
- Salt Formation & Complexation: Converting this compound into salts and forming inclusion complexes with cyclodextrins like sulfobutyl ether7 β-cyclodextrin (Captisol) can enhance its solubility and dissolution rate. []
Q13: What analytical methods are commonly used to quantify this compound and its metabolites in biological samples?
A13: Several techniques are utilized for this compound quantification:
- High-Performance Liquid Chromatography (HPLC): HPLC, coupled with UV [] or fluorescence detection [], is frequently employed for sensitive and specific analysis of this compound in biological matrices.
- Liquid Chromatography-Mass Spectrometry (LC-MS/MS): This highly sensitive and specific technique allows for simultaneous quantification of this compound and its metabolites, even at low concentrations. []
- Bioassay: While less common, bioassays can be used to assess the overall antifungal activity of serum samples from this compound-treated individuals. []
Disclaimer and Information on In-Vitro Research Products
Please be aware that all articles and product information presented on BenchChem are intended solely for informational purposes. The products available for purchase on BenchChem are specifically designed for in-vitro studies, which are conducted outside of living organisms. In-vitro studies, derived from the Latin term "in glass," involve experiments performed in controlled laboratory settings using cells or tissues. It is important to note that these products are not categorized as medicines or drugs, and they have not received approval from the FDA for the prevention, treatment, or cure of any medical condition, ailment, or disease. We must emphasize that any form of bodily introduction of these products into humans or animals is strictly prohibited by law. It is essential to adhere to these guidelines to ensure compliance with legal and ethical standards in research and experimentation.