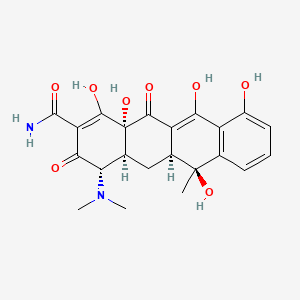
Tetracycline
Overview
Description
Tetracycline is a broad-spectrum antibiotic belonging to the tetracyclines family, which was discovered in the 1940s. It is used to treat a variety of infections caused by bacteria, including acne, cholera, brucellosis, plague, malaria, and syphilis . This compound works by inhibiting protein synthesis in bacteria, making it an effective bacteriostatic agent .
Mechanism of Action
Target of Action
Tetracycline, a broad-spectrum polyketide antibiotic, primarily targets the bacterial 30S ribosomal subunit . This subunit plays a crucial role in protein synthesis within the bacterial cell . This compound also binds to some extent to the bacterial 50S ribosomal subunit .
Mode of Action
This compound exerts its bacteriostatic effect by binding reversibly to the bacterial 30S ribosomal subunit . This binding prevents the incoming aminoacyl tRNA from attaching to the ribosome acceptor site, thereby interfering with protein synthesis . In addition, this compound may alter the cytoplasmic membrane, causing intracellular components to leak from bacterial cells .
Biochemical Pathways
The primary biochemical pathway affected by this compound is protein synthesis. By binding to the 30S ribosomal subunit, this compound inhibits the growth of the peptide chain, disrupting the protein synthesis process . This disruption affects various downstream effects, including the growth and replication of bacteria .
Pharmacokinetics
Tetracyclines, including this compound, can be categorized into three groups based on their pharmacokinetic properties . This compound belongs to the oldest group (Group 1), characterized by poor absorption after food intake . The pharmacokinetics of this compound involve absorption, distribution, metabolism, and excretion (ADME). The specifics of these processes can vary depending on factors such as dosage, route of administration, and individual patient characteristics .
Result of Action
The primary result of this compound’s action is the inhibition of bacterial growth, making it a bacteriostatic antibiotic . By preventing protein synthesis, this compound disrupts essential biological processes within the bacterial cell, leading to a halt in bacterial growth and replication . This effect is seen in a wide range of gram-positive and gram-negative bacteria, as well as several intracellular bacterial pathogens .
Action Environment
The action of this compound can be influenced by various environmental factors. For instance, the presence of this compound in the environment can lead to the development of antibiotic-resistant microorganisms . This resistance can pose a significant challenge to the efficacy of this compound and other antibiotics . Furthermore, the environmental presence of this compound can have detrimental effects on aquatic and terrestrial biodiversity . Therefore, understanding and managing the environmental impact of this compound is crucial for maintaining its efficacy and minimizing ecological harm .
Biochemical Analysis
Biochemical Properties
Tetracycline plays a crucial role in biochemical reactions by inhibiting the synthesis of proteins in bacterial cells. It interacts with the 30S ribosomal subunit, preventing the attachment of aminoacyl-tRNA to the mRNA-ribosome complex . This interaction is reversible and leads to a bacteriostatic effect, meaning it stops bacteria from multiplying without necessarily killing them. This compound also binds to the 50S ribosomal subunit to a lesser extent, which may alter the cytoplasmic membrane and cause intracellular components to leak . The compound’s ability to inhibit protein synthesis makes it effective against a wide range of bacterial species.
Cellular Effects
This compound affects various types of cells and cellular processes. In bacterial cells, it inhibits protein synthesis by binding to the 30S ribosomal subunit, which blocks the binding of aminoacyl-tRNA to the ribosome . This inhibition disrupts cell function and prevents the bacteria from growing and reproducing. In eukaryotic cells, this compound interacts weakly with the 80S ribosome, leading to a relatively weak inhibition of protein synthesis . This selective inhibition is why this compound is effective against bacteria while having limited side effects on human cells.
Molecular Mechanism
The molecular mechanism of this compound involves its binding to the 30S ribosomal subunit in bacterial cells. This binding prevents the attachment of aminoacyl-tRNA to the ribosome, thereby inhibiting protein synthesis . This compound’s action is bacteriostatic, meaning it stops bacteria from multiplying without killing them. The compound also binds to the 50S ribosomal subunit to a lesser extent, which may alter the cytoplasmic membrane and cause intracellular components to leak . This dual binding mechanism makes this compound a potent inhibitor of bacterial growth.
Temporal Effects in Laboratory Settings
In laboratory settings, the effects of this compound can change over time. The compound is stable as a dry powder but can degrade in aqueous solutions, especially at higher pH levels . Over time, bacteria can develop resistance to this compound, often through the acquisition of genes that code for efflux pumps or ribosomal protection proteins . These resistance mechanisms can reduce the effectiveness of this compound in long-term studies. Additionally, this compound’s bacteriostatic effect means that its impact on bacterial growth can be observed over several hours to days, depending on the experimental conditions.
Dosage Effects in Animal Models
The effects of this compound vary with different dosages in animal models. At therapeutic doses, this compound effectively inhibits bacterial growth without causing significant toxicity . At higher doses, this compound can cause adverse effects such as gastrointestinal disturbances, hepatotoxicity, and photosensitivity . In some animal models, high doses of this compound have been associated with changes in bone and teeth development due to its ability to bind to calcium . These dosage-dependent effects highlight the importance of careful dosing in both clinical and research settings.
Metabolic Pathways
This compound is metabolized in the liver and excreted primarily through the kidneys . The compound undergoes various metabolic transformations, including demethylation, deamination, and hydroxylation . These metabolic pathways are facilitated by enzymes such as cytochrome P450 oxidases. The metabolites of this compound can retain some antimicrobial activity, but they are generally less potent than the parent compound . Understanding these metabolic pathways is crucial for optimizing the use of this compound in clinical settings.
Transport and Distribution
This compound is transported and distributed within cells and tissues through both passive diffusion and active transport mechanisms . The compound can bind to plasma proteins, which facilitates its distribution throughout the body . This compound is also known to accumulate in certain tissues, such as the liver, kidneys, and bones . This tissue distribution is influenced by factors such as blood flow, tissue permeability, and the presence of binding proteins.
Subcellular Localization
Within cells, this compound localizes primarily to the cytoplasm, where it exerts its inhibitory effects on protein synthesis . The compound can also accumulate in the endoplasmic reticulum and other organelles involved in protein synthesis . This compound’s subcellular localization is influenced by its ability to bind to ribosomes and other cellular components. This localization is crucial for its effectiveness as an antibiotic, as it ensures that the compound reaches its target sites within bacterial cells.
Preparation Methods
Synthetic Routes and Reaction Conditions: Tetracycline can be synthesized through various methods. One common method involves the fermentation of Streptomyces bacteria, which naturally produce this compound. The process includes the extraction and purification of the antibiotic from the bacterial culture .
Industrial Production Methods: In industrial settings, this compound is often produced semi-synthetically. The process begins with the fermentation of Streptomyces bacteria to produce chlorthis compound, which is then chemically modified to produce this compound. This method allows for large-scale production of the antibiotic .
Chemical Reactions Analysis
Types of Reactions: Tetracycline undergoes various chemical reactions, including oxidation, reduction, and substitution reactions. These reactions can alter the chemical structure and properties of this compound, affecting its efficacy and stability .
Common Reagents and Conditions:
Oxidation: this compound can be oxidized using reagents such as hydrogen peroxide or potassium permanganate under acidic conditions.
Reduction: Reduction of this compound can be achieved using reagents like sodium borohydride or lithium aluminum hydride.
Major Products Formed: The major products formed from these reactions include various this compound derivatives, which may have different pharmacological properties and applications .
Scientific Research Applications
Tetracycline has a wide range of scientific research applications:
Chemistry: this compound is used as a model compound in studies of antibiotic synthesis and degradation.
Biology: It is used to study bacterial resistance mechanisms and the effects of antibiotics on microbial communities.
Medicine: this compound is used to treat bacterial infections and is also studied for its potential use in treating other conditions, such as cancer and inflammatory diseases.
Industry: this compound is used in animal husbandry to promote growth and prevent infections in livestock
Comparison with Similar Compounds
- Doxycycline
- Minocycline
- Tigecycline
- Omadacycline
- Eravacycline
Comparison: Tetracycline and its similar compounds share a common mechanism of action but differ in their pharmacokinetic properties and spectrum of activity. For example, doxycycline has a longer half-life and better absorption compared to this compound, making it more effective for certain infections. Minocycline has better penetration into tissues, while tigecycline is effective against a broader range of resistant bacteria .
This compound remains a valuable antibiotic due to its broad-spectrum activity and relatively low cost. the development of resistance has limited its use, prompting the development of newer this compound derivatives with improved properties .
Properties
IUPAC Name |
4-(dimethylamino)-1,6,10,11,12a-pentahydroxy-6-methyl-3,12-dioxo-4,4a,5,5a-tetrahydrotetracene-2-carboxamide | |
---|---|---|
Details | Computed by Lexichem TK 2.7.0 (PubChem release 2021.10.14) | |
Source | PubChem | |
URL | https://pubchem.ncbi.nlm.nih.gov | |
Description | Data deposited in or computed by PubChem | |
InChI |
InChI=1S/C22H24N2O8/c1-21(31)8-5-4-6-11(25)12(8)16(26)13-9(21)7-10-15(24(2)3)17(27)14(20(23)30)19(29)22(10,32)18(13)28/h4-6,9-10,15,25-26,29,31-32H,7H2,1-3H3,(H2,23,30) | |
Details | Computed by InChI 1.0.6 (PubChem release 2021.10.14) | |
Source | PubChem | |
URL | https://pubchem.ncbi.nlm.nih.gov | |
Description | Data deposited in or computed by PubChem | |
InChI Key |
NWXMGUDVXFXRIG-UHFFFAOYSA-N | |
Details | Computed by InChI 1.0.6 (PubChem release 2021.10.14) | |
Source | PubChem | |
URL | https://pubchem.ncbi.nlm.nih.gov | |
Description | Data deposited in or computed by PubChem | |
Canonical SMILES |
CC1(C2CC3C(C(=O)C(=C(C3(C(=O)C2=C(C4=C1C=CC=C4O)O)O)O)C(=O)N)N(C)C)O | |
Details | Computed by OEChem 2.3.0 (PubChem release 2021.10.14) | |
Source | PubChem | |
URL | https://pubchem.ncbi.nlm.nih.gov | |
Description | Data deposited in or computed by PubChem | |
Molecular Formula |
C22H24N2O8 | |
Details | Computed by PubChem 2.2 (PubChem release 2021.10.14) | |
Source | PubChem | |
URL | https://pubchem.ncbi.nlm.nih.gov | |
Description | Data deposited in or computed by PubChem | |
Molecular Weight |
444.4 g/mol | |
Details | Computed by PubChem 2.2 (PubChem release 2021.10.14) | |
Source | PubChem | |
URL | https://pubchem.ncbi.nlm.nih.gov | |
Description | Data deposited in or computed by PubChem | |
CAS No. |
60-54-8 | |
Record name | tetracycline | |
Source | DTP/NCI | |
URL | https://dtp.cancer.gov/dtpstandard/servlet/dwindex?searchtype=NSC&outputformat=html&searchlist=108579 | |
Description | The NCI Development Therapeutics Program (DTP) provides services and resources to the academic and private-sector research communities worldwide to facilitate the discovery and development of new cancer therapeutic agents. | |
Explanation | Unless otherwise indicated, all text within NCI products is free of copyright and may be reused without our permission. Credit the National Cancer Institute as the source. | |
Q1: How does tetracycline interact with its target and what are the downstream effects?
A1: this compound exerts its antibacterial activity by binding to the bacterial ribosome, specifically at the 30S ribosomal subunit [, ]. This binding interferes with the binding of aminoacyl-tRNA to the ribosomal acceptor (A) site, thereby inhibiting protein synthesis and halting bacterial growth [, ].
Q2: Can you provide some structural characterization of this compound?
A2: this compound is a yellow, crystalline compound with the molecular formula C22H24N2O8 and a molecular weight of 444.44 g/mol. The molecule features a tetracyclic naphtacene carboxamide ring system [, ]. Spectroscopic studies, including FT-IR and UV-Vis, have been employed to characterize the compound and investigate potential changes in its structural properties upon various treatments [].
Q3: How does the structure of this compound relate to its activity?
A3: The carboxamide group at the C2 position of the this compound molecule is essential for its transport into bacterial cells []. Modifications to the this compound structure can significantly alter its activity, potency, and selectivity [].
Q4: Are there any studies on the stability of this compound?
A4: Yes, studies have explored the stability of this compound hydrochloride under various conditions. One study investigated the effect of biofield treatment on chloramphenicol and this compound hydrochloride using FT-IR spectroscopy, suggesting that the treatment might enhance the chemical stability of both drugs []. Another study examined the release kinetics of 25% this compound hydrochloride-loaded ethylene vinyl acetate fibers, revealing a considerable decrease in the amount of biologically active this compound remaining in the fibers over time [].
Q5: Are there alternative compounds to this compound?
A5: Yes, other antibiotics, like macrolides and quinolones, can be used as alternatives to this compound []. The choice of treatment depends on factors such as the specific bacterial infection, resistance patterns, and patient-specific considerations [, ].
Q6: What analytical methods are employed to study this compound?
A6: Various analytical methods are used to characterize, quantify, and monitor this compound. These include:
- High-Performance Liquid Chromatography (HPLC): This method is commonly used to separate and quantify this compound and its analogs in various matrices, including honey, milk, and pharmaceutical formulations [, , , ].
- Liquid Chromatography-Mass Spectrometry (LC-MS/MS): This technique offers high sensitivity and selectivity for the determination of this compound residues in complex matrices like honey [].
- Ultra-Performance Liquid Chromatography (UPLC): This method provides rapid and efficient separation of this compound for analysis, as demonstrated in a study evaluating water-flow variation and this compound hydrochloride administration in swine [].
- Spectrophotometry: This method, often coupled with derivatization techniques like diazotization, can be used to quantify this compound hydrochloride in pharmaceutical formulations [].
- Agar Diffusion Inhibition Assay: This microbiological method assesses the biological activity of this compound, for example, in drug-loaded fibers [].
Q7: What are the mechanisms of resistance to this compound?
A7: Bacteria can develop resistance to this compound through several mechanisms:
- Efflux Pumps: Bacteria can acquire genes encoding efflux pumps, such as TetA(P) from Clostridium perfringens, which actively transport this compound out of the cell, reducing its intracellular concentration [].
- Ribosome Protection Proteins: These proteins, like TetM and TetO, can bind to the ribosome and displace this compound from its binding site, allowing protein synthesis to continue [].
- Mutations in Ribosomal RNA: Mutations in the 16S rRNA genes, particularly in the primary this compound binding pocket (Tet-1 site), can reduce the binding affinity of this compound to the ribosome, conferring decreased susceptibility [].
Q8: How prevalent is this compound resistance?
A8: The prevalence of this compound resistance varies depending on the bacterial species, geographic location, and antibiotic usage patterns. Studies have reported a high prevalence of this compound resistance genes, such as tetA and tetB, in Escherichia coli isolates from broiler chickens []. Additionally, resistance to this compound is a concern in Staphylococcus aureus [].
Q9: Are there any strategies to overcome this compound resistance?
A9: Researchers are exploring various strategies to combat this compound resistance:
- Combination Therapy: Using this compound in combination with other antibiotics, such as rifampicin, can enhance its activity against resistant strains [].
- Drug Delivery Systems: Novel drug delivery systems, such as encapsulation in cyclodextrins, are being explored to improve the bioavailability and efficacy of this compound [].
Q10: What is the environmental impact of this compound?
A10: this compound, like other antibiotics, can enter the environment through wastewater and agricultural runoff, potentially contributing to the spread of antibiotic resistance genes []. Studies have investigated the use of UV light disinfection to degrade this compound in wastewater effluents []. Other research focuses on using porous graphitic biochar for the adsorption and removal of this compound from aqueous solutions [].
Disclaimer and Information on In-Vitro Research Products
Please be aware that all articles and product information presented on BenchChem are intended solely for informational purposes. The products available for purchase on BenchChem are specifically designed for in-vitro studies, which are conducted outside of living organisms. In-vitro studies, derived from the Latin term "in glass," involve experiments performed in controlled laboratory settings using cells or tissues. It is important to note that these products are not categorized as medicines or drugs, and they have not received approval from the FDA for the prevention, treatment, or cure of any medical condition, ailment, or disease. We must emphasize that any form of bodily introduction of these products into humans or animals is strictly prohibited by law. It is essential to adhere to these guidelines to ensure compliance with legal and ethical standards in research and experimentation.