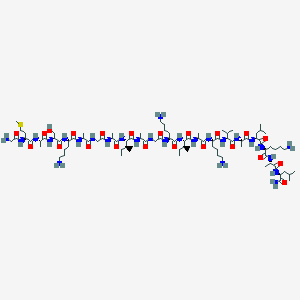
聚乳酸-共-乙醇酸 (PLGA)
描述
PGLa is a 21-residue peptide known for its antimicrobial activity . It is an amphiphilic α-helical membranolytic peptide from frog skin . PGLa is a copolymer of glycolide and L-lactide, abbreviated to PGLA . It is used in many long-acting drug formulations approved by the US Food and Drug Administration (FDA) .
Synthesis Analysis
PGLa is synthesized by a catalyst-mediated random ring-opening copolymerization of lactic acid and glycolic acid where both polymers link together through an ester linkage . The copolymerization of glycolide and L-lactide forms PGLa .
Molecular Structure Analysis
The PGLa peptide is known to bind to a model anionic DMPC/DMPG bilayer at a high peptide:lipid ratio that promotes PGLa dimerization . The increase in the peptide:lipid ratio suppresses PGLa helical propensity, tilts the bound peptide toward the bilayer hydrophobic core, and forces it deeper into the bilayer .
Chemical Reactions Analysis
PGLa peptides are known to interact with anionic lipid bilayers . At a high peptide:lipid ratio, PGLa binding induces weaker bilayer thinning but deeper water permeation .
Physical and Chemical Properties Analysis
PGLa is a biocompatible and biodegradable polymer . It has been used in many long-acting drug formulations .
科学研究应用
聚乳酸-共-乙醇酸 (PLGA) (Gla 肽) 在科学研究中的应用:PLGA,也称为 Gla 肽或 PGLa,是一种在科学研究中具有多种应用的化合物。以下是六种独特的应用,每种应用都在其自己的部分中详细介绍。
抗生素特性
PLGA 被鉴定为一种在青蛙皮肤中发现的抗生素肽。它属于蛙皮素家族,在膜结合后可以形成两亲性 α-螺旋结构。 其阳离子性质使其对细菌膜具有高亲和力,使其能够有效地渗透细菌膜 .
癌症治疗
PLGA 基纳米粒子 (NPs) 用于癌症治疗中的靶向药物递送、基因治疗、热疗和光动力治疗。 这些 NPs 可以被设计为靶向体内特定部位,确保治疗剂的安全有效递送 .
药物递送系统
PLGA 聚合物广泛用于医疗应用中的药物递送。 它们可变的熔点和结晶度允许创建能够以受控方式递送药物的微球和纳米粒子 .
基因治疗
PLGA 纳米粒子已被证明可以有效地将 siRNA 递送至细胞质,这对于基因治疗应用至关重要。 这些纳米粒子的形状,例如针状 NPs,会影响其穿越细胞膜的效率 .
抗菌研究
关于 PGLa 肽的研究包括研究它们在阴离子脂质双层中的结合和二聚化,这对于理解它们的抗菌特性和对抗抗生素耐药细菌的潜在应用非常重要 .
生物医学工程
PLA 和 PLGA 的生物相容性和生物降解特性使其适用于各种生物医学应用,包括开发用于组织工程的支架 .
酶生产
PLGA 与从芽孢杆菌属等细菌菌株中重组生产酶(如 β-1,3-葡聚糖酶)有关,这在农业和工业中具有潜在应用 .
CRISPR-Cas9 递送
PLGA 纳米粒子已被探索为将 CRISPR-Cas9 系统递送至细胞以进行基因编辑的载体。 这种方法对未来基因治疗的体内应用显示出前景 .
这些领域中的每一个都展示了 PLGA 在跨越各个领域的科学研究进步中的多功能性和潜力。
GenScript - PGLa <svg class="icon" height="16" p-id="1735" t="1709264788668" version="1.1" viewbox="0 0 1024 102
作用机制
- The exact cytotoxic mechanism remains to be fully elucidated, but pore formation is a likely contributor .
Mode of Action
Biochemical Pathways
Result of Action
未来方向
生化分析
Biochemical Properties
PGLA interacts with various biomolecules, playing a significant role in biochemical reactions . It specifically hydrolyzes β-1,3-glucan . The presence of non-catalytic modules in PGLA influences its enzymatic properties . The N-and C-terminal domains of PGLA exhibit strong binding activity towards insoluble β-1,3-glucan, enhancing its hydrolysis .
Cellular Effects
PGLA has notable effects on various types of cells and cellular processes . For instance, PGLA-loaded TiO2 nanotube arrays have shown promising antibacterial and anti-inflammatory properties in osteoblast-like MG-63 cells . PGLA also influences cell function by affecting cell adhesion, proliferation, and osteogenic activity .
Molecular Mechanism
The molecular mechanism of PGLA involves its ability to compromise bacterial membranes, contributing to its antimicrobial activity . A proposed model suggests the formation of a membrane-spanning antiparallel PGLA dimer, stabilized by Gly-Gly contacts, where each PGLA monomer interacts with one MAG2 molecule at its C-terminus .
Temporal Effects in Laboratory Settings
The effects of PGLA change over time in laboratory settings. The degradation of PGLA is related to the ratio of the monomers used in its production . The higher the content of glycolide units, the lower the time required for degradation .
Dosage Effects in Animal Models
The effects of PGLA vary with different dosages in animal models . PLGA-based nanoparticles show promise in releasing different drugs, proteins, and nucleic acids in a stable and controlled manner, greatly ameliorating their therapeutic efficacy .
Metabolic Pathways
PGLA is involved in metabolic pathways that lead to the production of the original monomers: lactic acid and glycolic acid . These monomers are by-products of various metabolic pathways in the body. Lactic acid is metabolized in the tricarboxylic acid cycle and eliminated via carbon dioxide and water .
Transport and Distribution
PGLA is transported and distributed within cells and tissues . PLGA-based nanoparticles improve the pharmacokinetic and pharmacodynamic properties of drugs, reduce side effects, improve stability, prolong the release of the drug, and reduce the dosing frequency .
Subcellular Localization
Current studies are leveraging machine learning tools to predict multi-label subcellular localization . These predictions can provide insights into the activity and function of PGLA at the subcellular level .
属性
IUPAC Name |
(2S)-6-amino-2-[[2-[[(2S)-2-[[(2S,3S)-2-[[(2S)-2-[[2-[[(2S)-2-[[(2S)-6-amino-2-[[(2S)-2-[[(2S)-2-[[(2S)-2-[(2-aminoacetyl)amino]-4-methylsulfanylbutanoyl]amino]propanoyl]amino]-3-hydroxypropanoyl]amino]hexanoyl]amino]propanoyl]amino]acetyl]amino]propanoyl]amino]-3-methylpentanoyl]amino]propanoyl]amino]acetyl]amino]-N-[(2S,3S)-1-[[(2S)-1-[[(2S)-6-amino-1-[[(2S)-1-[[(2S)-1-[[(2S)-1-[[(2S)-6-amino-1-[[(2S)-1-[[(2S)-1-amino-4-methyl-1-oxopentan-2-yl]amino]-1-oxopropan-2-yl]amino]-1-oxohexan-2-yl]amino]-4-methyl-1-oxopentan-2-yl]amino]-1-oxopropan-2-yl]amino]-3-methyl-1-oxobutan-2-yl]amino]-1-oxohexan-2-yl]amino]-1-oxopropan-2-yl]amino]-3-methyl-1-oxopentan-2-yl]hexanamide | |
---|---|---|
Source | PubChem | |
URL | https://pubchem.ncbi.nlm.nih.gov | |
Description | Data deposited in or computed by PubChem | |
InChI |
InChI=1S/C88H162N26O22S/c1-19-48(9)69(113-78(126)52(13)97-66(117)42-95-72(120)50(11)98-79(127)59(31-23-27-36-91)108-85(133)64(44-115)111-77(125)54(15)100-81(129)61(33-38-137-18)104-65(116)41-93)87(135)101-51(12)73(121)96-43-67(118)105-57(29-21-25-34-89)82(130)114-70(49(10)20-2)88(136)103-55(16)74(122)106-60(32-24-28-37-92)83(131)112-68(47(7)8)86(134)102-56(17)76(124)110-63(40-46(5)6)84(132)107-58(30-22-26-35-90)80(128)99-53(14)75(123)109-62(71(94)119)39-45(3)4/h45-64,68-70,115H,19-44,89-93H2,1-18H3,(H2,94,119)(H,95,120)(H,96,121)(H,97,117)(H,98,127)(H,99,128)(H,100,129)(H,101,135)(H,102,134)(H,103,136)(H,104,116)(H,105,118)(H,106,122)(H,107,132)(H,108,133)(H,109,123)(H,110,124)(H,111,125)(H,112,131)(H,113,126)(H,114,130)/t48-,49-,50-,51-,52-,53-,54-,55-,56-,57-,58-,59-,60-,61-,62-,63-,64-,68-,69-,70-/m0/s1 | |
Source | PubChem | |
URL | https://pubchem.ncbi.nlm.nih.gov | |
Description | Data deposited in or computed by PubChem | |
InChI Key |
PJRSUKFWFKUDTH-JWDJOUOUSA-N | |
Source | PubChem | |
URL | https://pubchem.ncbi.nlm.nih.gov | |
Description | Data deposited in or computed by PubChem | |
Canonical SMILES |
CCC(C)C(C(=O)NC(C)C(=O)NCC(=O)NC(CCCCN)C(=O)NC(C(C)CC)C(=O)NC(C)C(=O)NC(CCCCN)C(=O)NC(C(C)C)C(=O)NC(C)C(=O)NC(CC(C)C)C(=O)NC(CCCCN)C(=O)NC(C)C(=O)NC(CC(C)C)C(=O)N)NC(=O)C(C)NC(=O)CNC(=O)C(C)NC(=O)C(CCCCN)NC(=O)C(CO)NC(=O)C(C)NC(=O)C(CCSC)NC(=O)CN | |
Source | PubChem | |
URL | https://pubchem.ncbi.nlm.nih.gov | |
Description | Data deposited in or computed by PubChem | |
Isomeric SMILES |
CC[C@H](C)[C@@H](C(=O)N[C@@H](C)C(=O)NCC(=O)N[C@@H](CCCCN)C(=O)N[C@@H]([C@@H](C)CC)C(=O)N[C@@H](C)C(=O)N[C@@H](CCCCN)C(=O)N[C@@H](C(C)C)C(=O)N[C@@H](C)C(=O)N[C@@H](CC(C)C)C(=O)N[C@@H](CCCCN)C(=O)N[C@@H](C)C(=O)N[C@@H](CC(C)C)C(=O)N)NC(=O)[C@H](C)NC(=O)CNC(=O)[C@H](C)NC(=O)[C@H](CCCCN)NC(=O)[C@H](CO)NC(=O)[C@H](C)NC(=O)[C@H](CCSC)NC(=O)CN | |
Source | PubChem | |
URL | https://pubchem.ncbi.nlm.nih.gov | |
Description | Data deposited in or computed by PubChem | |
Molecular Formula |
C88H162N26O22S | |
Source | PubChem | |
URL | https://pubchem.ncbi.nlm.nih.gov | |
Description | Data deposited in or computed by PubChem | |
Molecular Weight |
1968.5 g/mol | |
Source | PubChem | |
URL | https://pubchem.ncbi.nlm.nih.gov | |
Description | Data deposited in or computed by PubChem | |
CAS No. |
102068-15-5 | |
Record name | Peptide-gly-leu-amide | |
Source | ChemIDplus | |
URL | https://pubchem.ncbi.nlm.nih.gov/substance/?source=chemidplus&sourceid=0102068155 | |
Description | ChemIDplus is a free, web search system that provides access to the structure and nomenclature authority files used for the identification of chemical substances cited in National Library of Medicine (NLM) databases, including the TOXNET system. | |
Q1: How does PGLa interact with bacterial membranes?
A1: PGLa, due to its amphipathic nature, interacts preferentially with negatively charged bacterial membranes [, , ]. At low concentrations, it lies flat on the membrane surface (S-state) []. At higher concentrations, it tilts relative to the membrane normal (T-state) and forms dimeric structures, promoting membrane permeabilization [, ].
Q2: Does PGLa form pores in bacterial membranes?
A2: Yes, PGLa induces the formation of toroidal pores in bacterial membranes. These pores mediate the transport of ions, small molecules, and even the peptide itself across the membrane, ultimately leading to cell death [, ].
Q3: How does the transmembrane potential (TMP) affect PGLa's activity?
A3: Interestingly, higher TMP increases the population of PGLa in the tilted (T-state) orientation []. This positive feedback loop between TMP and PGLa tilt angle, driven by electrostatic interactions, likely contributes to its antimicrobial activity [].
Q4: Does PGLa exhibit synergistic activity with other antimicrobial peptides?
A4: Yes, PGLa demonstrates remarkable synergism with magainin 2, another antimicrobial peptide [, ]. They form a 1:1 complex within the membrane, significantly enhancing pore formation and membrane permeabilization compared to individual peptides [, ].
Q5: What spectroscopic techniques are used to study PGLa's structure and interactions with membranes?
A6: Circular dichroism (CD) spectroscopy is commonly used to determine PGLa's secondary structure, revealing its transition from random coil in solution to α-helix upon membrane binding [, ]. Solid-state NMR, particularly with ¹⁹F and ²H labeling, provides detailed insights into PGLa's orientation and dynamics within lipid bilayers [, ].
Q6: How does PGLa's structure influence its stability and aggregation behavior?
A7: PGLa's amphipathic α-helical structure contributes to its self-assembly and membrane interaction properties [, ]. The balance between hydrophobic and electrostatic interactions influences its stability and aggregation in solution and membrane environments [, ].
Q7: Can PGLa be incorporated into biomaterials for biomedical applications?
A8: Research suggests the potential of incorporating PGLa into biomaterials. For instance, chitosan-coated PGLA brachytherapy seed carriers incorporating PGLa showed promising biocompatibility and controlled degradation properties in in vivo studies [, ].
Q8: Does PGLa possess any catalytic activity?
A8: PGLa is primarily known for its antimicrobial activity, not its catalytic properties. Its mode of action involves membrane disruption rather than enzymatic activity.
Q9: How is computational chemistry used to study PGLa's interactions with membranes?
A10: Molecular dynamics (MD) simulations are extensively used to investigate PGLa's membrane binding mechanism, providing atomic-level insights into its interactions with lipid molecules [, , , ]. These simulations help visualize its insertion, orientation, and pore formation within different membrane environments [, , , ].
Q10: Have any QSAR models been developed for PGLa and its analogs?
A11: QSAR models, correlating PGLa's structure to its antimicrobial activity, are actively being explored. These models could aid in designing more potent and selective antimicrobial peptides based on PGLa's scaffold [].
Q11: How do modifications to PGLa's amino acid sequence affect its activity?
A12: Studies altering PGLa's sequence, especially involving hydrophobic residues and charges, have shown significant effects on its membrane binding, pore formation, hemolytic activity, and antimicrobial potency [, ]. These findings highlight the delicate balance required for optimal activity and selectivity [, ].
Q12: Are there specific SHE regulations regarding PGLa research and development?
A12: As a peptide with potential therapeutic applications, PGLa research and development fall under general guidelines for safe handling of biological materials and pharmaceuticals. Researchers adhere to established laboratory safety procedures and ethical considerations.
Q13: What in vitro models are used to assess PGLa's antimicrobial activity?
A16: PGLa's efficacy is commonly evaluated in vitro using bacterial growth inhibition assays, determining its minimum inhibitory concentration (MIC) against various bacterial strains [, ]. Additionally, liposome leakage assays are employed to assess its membrane permeabilization activity [, ].
Q14: Has PGLa been tested in animal models of bacterial infection?
A17: While in vivo studies specifically focusing on PGLa's efficacy against bacterial infections are limited within the provided research, its potential in biomedical applications, as demonstrated by its incorporation into biocompatible materials, suggests a promising avenue for future investigations [, ].
体外研究产品的免责声明和信息
请注意,BenchChem 上展示的所有文章和产品信息仅供信息参考。 BenchChem 上可购买的产品专为体外研究设计,这些研究在生物体外进行。体外研究,源自拉丁语 "in glass",涉及在受控实验室环境中使用细胞或组织进行的实验。重要的是要注意,这些产品没有被归类为药物或药品,他们没有得到 FDA 的批准,用于预防、治疗或治愈任何医疗状况、疾病或疾病。我们必须强调,将这些产品以任何形式引入人类或动物的身体都是法律严格禁止的。遵守这些指南对确保研究和实验的法律和道德标准的符合性至关重要。