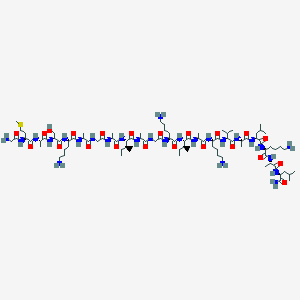
ポリ乳酸-グリコール酸共重合体 (PLGA)
説明
PGLa is a 21-residue peptide known for its antimicrobial activity . It is an amphiphilic α-helical membranolytic peptide from frog skin . PGLa is a copolymer of glycolide and L-lactide, abbreviated to PGLA . It is used in many long-acting drug formulations approved by the US Food and Drug Administration (FDA) .
Synthesis Analysis
PGLa is synthesized by a catalyst-mediated random ring-opening copolymerization of lactic acid and glycolic acid where both polymers link together through an ester linkage . The copolymerization of glycolide and L-lactide forms PGLa .
Molecular Structure Analysis
The PGLa peptide is known to bind to a model anionic DMPC/DMPG bilayer at a high peptide:lipid ratio that promotes PGLa dimerization . The increase in the peptide:lipid ratio suppresses PGLa helical propensity, tilts the bound peptide toward the bilayer hydrophobic core, and forces it deeper into the bilayer .
Chemical Reactions Analysis
PGLa peptides are known to interact with anionic lipid bilayers . At a high peptide:lipid ratio, PGLa binding induces weaker bilayer thinning but deeper water permeation .
Physical and Chemical Properties Analysis
PGLa is a biocompatible and biodegradable polymer . It has been used in many long-acting drug formulations .
科学的研究の応用
ポリ乳酸-グリコール酸共重合体 (PLGA) (Glaペプチド) の科学研究における用途: PLGAは、GlaペプチドまたはPGLaとしても知られており、科学研究においてさまざまな用途を持つ化合物です。以下は、それぞれ独自のセクションで詳しく説明されている6つのユニークな用途です。
抗生物質の性質
PLGAは、カエルの皮膚に見られる抗生物質ペプチドとして同定されています。それはマガイニンファミリーに属し、膜結合時に両親媒性α-ヘリックス構造をとることができます。 そのカチオン性の性質により、細菌膜に対する高い親和性を持ち、効果的に膜透過性を高めることができます .
がん治療
PLGAベースのナノ粒子(NPs)は、標的薬物送達、遺伝子治療、過熱療法、光線力学療法などの癌治療に使用されています。 これらのNPsは、体の特定の部位を標的にするように設計することができ、治療剤の安全で効果的な送達を保証します .
薬物送達システム
PLGAポリマーは、薬物送達のための医療用途で広く使用されています。 それらの可変的な融点と結晶化度により、薬物を制御された方法で送達できるマイクロカプセルやナノ粒子を作成できます .
遺伝子治療
PLGAナノ粒子は、遺伝子治療用途に不可欠な、siRNAを細胞質に効果的に送達することが示されています。 これらのナノ粒子の形状(針状のNPsなど)は、細胞膜を横切る効率に影響を与える可能性があります .
抗菌研究
PGLaペプチドに関する研究には、陰イオン性脂質二重層におけるその結合と二量体化の研究が含まれ、これはその抗菌特性と抗生物質耐性菌との闘いにおける潜在的な用途を理解するために重要です .
バイオメディカルエンジニアリング
PLAおよびPLGAの生体適合性と生分解性により、組織工学のための足場の開発など、さまざまなバイオメディカル用途に適しています .
酵素生産
PLGAは、農業や産業で潜在的な用途を持つ、Paenibacillus sp.などの細菌株からのβ-1,3-グルカナーゼなどの酵素の組換え生産に関与しています .
CRISPR-Cas9送達
PLGAナノ粒子は、遺伝子編集のために細胞にCRISPR-Cas9システムを導入するためのキャリアとして調査されています。 この方法は、将来の遺伝子治療のin vivoでの応用にとって有望です .
これらの分野はそれぞれ、さまざまな分野における科学研究の進歩におけるPLGAの汎用性と可能性を示しています。
GenScript - PGLa <a aria-label="2: GenScript - PGLa" data-citationid="a0ec35c0-6d53-782a-43ad-02b60288e7b8-41" h="ID=SERP,5015.1" href="https://aapsopen.springeropen.com/articles
作用機序
- The exact cytotoxic mechanism remains to be fully elucidated, but pore formation is a likely contributor .
Mode of Action
Biochemical Pathways
Result of Action
将来の方向性
生化学分析
Biochemical Properties
PGLA interacts with various biomolecules, playing a significant role in biochemical reactions . It specifically hydrolyzes β-1,3-glucan . The presence of non-catalytic modules in PGLA influences its enzymatic properties . The N-and C-terminal domains of PGLA exhibit strong binding activity towards insoluble β-1,3-glucan, enhancing its hydrolysis .
Cellular Effects
PGLA has notable effects on various types of cells and cellular processes . For instance, PGLA-loaded TiO2 nanotube arrays have shown promising antibacterial and anti-inflammatory properties in osteoblast-like MG-63 cells . PGLA also influences cell function by affecting cell adhesion, proliferation, and osteogenic activity .
Molecular Mechanism
The molecular mechanism of PGLA involves its ability to compromise bacterial membranes, contributing to its antimicrobial activity . A proposed model suggests the formation of a membrane-spanning antiparallel PGLA dimer, stabilized by Gly-Gly contacts, where each PGLA monomer interacts with one MAG2 molecule at its C-terminus .
Temporal Effects in Laboratory Settings
The effects of PGLA change over time in laboratory settings. The degradation of PGLA is related to the ratio of the monomers used in its production . The higher the content of glycolide units, the lower the time required for degradation .
Dosage Effects in Animal Models
The effects of PGLA vary with different dosages in animal models . PLGA-based nanoparticles show promise in releasing different drugs, proteins, and nucleic acids in a stable and controlled manner, greatly ameliorating their therapeutic efficacy .
Metabolic Pathways
PGLA is involved in metabolic pathways that lead to the production of the original monomers: lactic acid and glycolic acid . These monomers are by-products of various metabolic pathways in the body. Lactic acid is metabolized in the tricarboxylic acid cycle and eliminated via carbon dioxide and water .
Transport and Distribution
PGLA is transported and distributed within cells and tissues . PLGA-based nanoparticles improve the pharmacokinetic and pharmacodynamic properties of drugs, reduce side effects, improve stability, prolong the release of the drug, and reduce the dosing frequency .
Subcellular Localization
Current studies are leveraging machine learning tools to predict multi-label subcellular localization . These predictions can provide insights into the activity and function of PGLA at the subcellular level .
特性
IUPAC Name |
(2S)-6-amino-2-[[2-[[(2S)-2-[[(2S,3S)-2-[[(2S)-2-[[2-[[(2S)-2-[[(2S)-6-amino-2-[[(2S)-2-[[(2S)-2-[[(2S)-2-[(2-aminoacetyl)amino]-4-methylsulfanylbutanoyl]amino]propanoyl]amino]-3-hydroxypropanoyl]amino]hexanoyl]amino]propanoyl]amino]acetyl]amino]propanoyl]amino]-3-methylpentanoyl]amino]propanoyl]amino]acetyl]amino]-N-[(2S,3S)-1-[[(2S)-1-[[(2S)-6-amino-1-[[(2S)-1-[[(2S)-1-[[(2S)-1-[[(2S)-6-amino-1-[[(2S)-1-[[(2S)-1-amino-4-methyl-1-oxopentan-2-yl]amino]-1-oxopropan-2-yl]amino]-1-oxohexan-2-yl]amino]-4-methyl-1-oxopentan-2-yl]amino]-1-oxopropan-2-yl]amino]-3-methyl-1-oxobutan-2-yl]amino]-1-oxohexan-2-yl]amino]-1-oxopropan-2-yl]amino]-3-methyl-1-oxopentan-2-yl]hexanamide | |
---|---|---|
Source | PubChem | |
URL | https://pubchem.ncbi.nlm.nih.gov | |
Description | Data deposited in or computed by PubChem | |
InChI |
InChI=1S/C88H162N26O22S/c1-19-48(9)69(113-78(126)52(13)97-66(117)42-95-72(120)50(11)98-79(127)59(31-23-27-36-91)108-85(133)64(44-115)111-77(125)54(15)100-81(129)61(33-38-137-18)104-65(116)41-93)87(135)101-51(12)73(121)96-43-67(118)105-57(29-21-25-34-89)82(130)114-70(49(10)20-2)88(136)103-55(16)74(122)106-60(32-24-28-37-92)83(131)112-68(47(7)8)86(134)102-56(17)76(124)110-63(40-46(5)6)84(132)107-58(30-22-26-35-90)80(128)99-53(14)75(123)109-62(71(94)119)39-45(3)4/h45-64,68-70,115H,19-44,89-93H2,1-18H3,(H2,94,119)(H,95,120)(H,96,121)(H,97,117)(H,98,127)(H,99,128)(H,100,129)(H,101,135)(H,102,134)(H,103,136)(H,104,116)(H,105,118)(H,106,122)(H,107,132)(H,108,133)(H,109,123)(H,110,124)(H,111,125)(H,112,131)(H,113,126)(H,114,130)/t48-,49-,50-,51-,52-,53-,54-,55-,56-,57-,58-,59-,60-,61-,62-,63-,64-,68-,69-,70-/m0/s1 | |
Source | PubChem | |
URL | https://pubchem.ncbi.nlm.nih.gov | |
Description | Data deposited in or computed by PubChem | |
InChI Key |
PJRSUKFWFKUDTH-JWDJOUOUSA-N | |
Source | PubChem | |
URL | https://pubchem.ncbi.nlm.nih.gov | |
Description | Data deposited in or computed by PubChem | |
Canonical SMILES |
CCC(C)C(C(=O)NC(C)C(=O)NCC(=O)NC(CCCCN)C(=O)NC(C(C)CC)C(=O)NC(C)C(=O)NC(CCCCN)C(=O)NC(C(C)C)C(=O)NC(C)C(=O)NC(CC(C)C)C(=O)NC(CCCCN)C(=O)NC(C)C(=O)NC(CC(C)C)C(=O)N)NC(=O)C(C)NC(=O)CNC(=O)C(C)NC(=O)C(CCCCN)NC(=O)C(CO)NC(=O)C(C)NC(=O)C(CCSC)NC(=O)CN | |
Source | PubChem | |
URL | https://pubchem.ncbi.nlm.nih.gov | |
Description | Data deposited in or computed by PubChem | |
Isomeric SMILES |
CC[C@H](C)[C@@H](C(=O)N[C@@H](C)C(=O)NCC(=O)N[C@@H](CCCCN)C(=O)N[C@@H]([C@@H](C)CC)C(=O)N[C@@H](C)C(=O)N[C@@H](CCCCN)C(=O)N[C@@H](C(C)C)C(=O)N[C@@H](C)C(=O)N[C@@H](CC(C)C)C(=O)N[C@@H](CCCCN)C(=O)N[C@@H](C)C(=O)N[C@@H](CC(C)C)C(=O)N)NC(=O)[C@H](C)NC(=O)CNC(=O)[C@H](C)NC(=O)[C@H](CCCCN)NC(=O)[C@H](CO)NC(=O)[C@H](C)NC(=O)[C@H](CCSC)NC(=O)CN | |
Source | PubChem | |
URL | https://pubchem.ncbi.nlm.nih.gov | |
Description | Data deposited in or computed by PubChem | |
Molecular Formula |
C88H162N26O22S | |
Source | PubChem | |
URL | https://pubchem.ncbi.nlm.nih.gov | |
Description | Data deposited in or computed by PubChem | |
Molecular Weight |
1968.5 g/mol | |
Source | PubChem | |
URL | https://pubchem.ncbi.nlm.nih.gov | |
Description | Data deposited in or computed by PubChem | |
CAS No. |
102068-15-5 | |
Record name | Peptide-gly-leu-amide | |
Source | ChemIDplus | |
URL | https://pubchem.ncbi.nlm.nih.gov/substance/?source=chemidplus&sourceid=0102068155 | |
Description | ChemIDplus is a free, web search system that provides access to the structure and nomenclature authority files used for the identification of chemical substances cited in National Library of Medicine (NLM) databases, including the TOXNET system. | |
Q1: How does PGLa interact with bacterial membranes?
A1: PGLa, due to its amphipathic nature, interacts preferentially with negatively charged bacterial membranes [, , ]. At low concentrations, it lies flat on the membrane surface (S-state) []. At higher concentrations, it tilts relative to the membrane normal (T-state) and forms dimeric structures, promoting membrane permeabilization [, ].
Q2: Does PGLa form pores in bacterial membranes?
A2: Yes, PGLa induces the formation of toroidal pores in bacterial membranes. These pores mediate the transport of ions, small molecules, and even the peptide itself across the membrane, ultimately leading to cell death [, ].
Q3: How does the transmembrane potential (TMP) affect PGLa's activity?
A3: Interestingly, higher TMP increases the population of PGLa in the tilted (T-state) orientation []. This positive feedback loop between TMP and PGLa tilt angle, driven by electrostatic interactions, likely contributes to its antimicrobial activity [].
Q4: Does PGLa exhibit synergistic activity with other antimicrobial peptides?
A4: Yes, PGLa demonstrates remarkable synergism with magainin 2, another antimicrobial peptide [, ]. They form a 1:1 complex within the membrane, significantly enhancing pore formation and membrane permeabilization compared to individual peptides [, ].
Q5: What spectroscopic techniques are used to study PGLa's structure and interactions with membranes?
A6: Circular dichroism (CD) spectroscopy is commonly used to determine PGLa's secondary structure, revealing its transition from random coil in solution to α-helix upon membrane binding [, ]. Solid-state NMR, particularly with ¹⁹F and ²H labeling, provides detailed insights into PGLa's orientation and dynamics within lipid bilayers [, ].
Q6: How does PGLa's structure influence its stability and aggregation behavior?
A7: PGLa's amphipathic α-helical structure contributes to its self-assembly and membrane interaction properties [, ]. The balance between hydrophobic and electrostatic interactions influences its stability and aggregation in solution and membrane environments [, ].
Q7: Can PGLa be incorporated into biomaterials for biomedical applications?
A8: Research suggests the potential of incorporating PGLa into biomaterials. For instance, chitosan-coated PGLA brachytherapy seed carriers incorporating PGLa showed promising biocompatibility and controlled degradation properties in in vivo studies [, ].
Q8: Does PGLa possess any catalytic activity?
A8: PGLa is primarily known for its antimicrobial activity, not its catalytic properties. Its mode of action involves membrane disruption rather than enzymatic activity.
Q9: How is computational chemistry used to study PGLa's interactions with membranes?
A10: Molecular dynamics (MD) simulations are extensively used to investigate PGLa's membrane binding mechanism, providing atomic-level insights into its interactions with lipid molecules [, , , ]. These simulations help visualize its insertion, orientation, and pore formation within different membrane environments [, , , ].
Q10: Have any QSAR models been developed for PGLa and its analogs?
A11: QSAR models, correlating PGLa's structure to its antimicrobial activity, are actively being explored. These models could aid in designing more potent and selective antimicrobial peptides based on PGLa's scaffold [].
Q11: How do modifications to PGLa's amino acid sequence affect its activity?
A12: Studies altering PGLa's sequence, especially involving hydrophobic residues and charges, have shown significant effects on its membrane binding, pore formation, hemolytic activity, and antimicrobial potency [, ]. These findings highlight the delicate balance required for optimal activity and selectivity [, ].
Q12: Are there specific SHE regulations regarding PGLa research and development?
A12: As a peptide with potential therapeutic applications, PGLa research and development fall under general guidelines for safe handling of biological materials and pharmaceuticals. Researchers adhere to established laboratory safety procedures and ethical considerations.
Q13: What in vitro models are used to assess PGLa's antimicrobial activity?
A16: PGLa's efficacy is commonly evaluated in vitro using bacterial growth inhibition assays, determining its minimum inhibitory concentration (MIC) against various bacterial strains [, ]. Additionally, liposome leakage assays are employed to assess its membrane permeabilization activity [, ].
Q14: Has PGLa been tested in animal models of bacterial infection?
A17: While in vivo studies specifically focusing on PGLa's efficacy against bacterial infections are limited within the provided research, its potential in biomedical applications, as demonstrated by its incorporation into biocompatible materials, suggests a promising avenue for future investigations [, ].
試験管内研究製品の免責事項と情報
BenchChemで提示されるすべての記事および製品情報は、情報提供を目的としています。BenchChemで購入可能な製品は、生体外研究のために特別に設計されています。生体外研究は、ラテン語の "in glass" に由来し、生物体の外で行われる実験を指します。これらの製品は医薬品または薬として分類されておらず、FDAから任何の医療状態、病気、または疾患の予防、治療、または治癒のために承認されていません。これらの製品を人間または動物に体内に導入する形態は、法律により厳格に禁止されています。これらのガイドラインに従うことは、研究と実験において法的および倫理的な基準の遵守を確実にするために重要です。