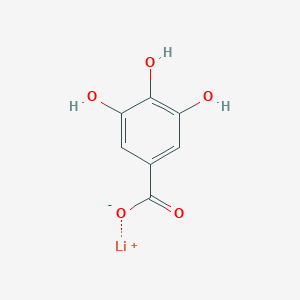
Lithium gallate
描述
Lithium gallate (LiGaO₂) is a wide-band-gap semiconductor with an optical gap greater than 5.3 eV . It is known for its broad functionality in optical devices that generate, detect, and process light across much of the ultraviolet spectral region . This compound is a ternary analog of zinc oxide, where half of the zinc ions are replaced by lithium ions and the other half by gallium ions in an ordered arrangement .
作用机制
Target of Action
Lithium Gallate, also known as lithium;3,4,5-trihydroxybenzoate, primarily targets neurotransmitters and second messenger systems in the brain . It interacts with dopamine and glutamate pathways, which play crucial roles in the pathogenesis of bipolar affective disorder . This compound also affects GABA pathways, an inhibitory transmitter that modulates glutamate and dopamine .
Mode of Action
This compound exerts its effects by competing for macromolecular sites that are relatively specific for other cations, especially sodium and magnesium . In the dopamine pathway, it decreases presynaptic dopamine activity and inactivates postsynaptic G protein, reducing excitatory neurotransmission in the brain . In the glutamate pathway, it enhances inhibitory neurotransmission by downregulating the NMDA receptor and inhibiting the myoinositol second messenger system directly .
Biochemical Pathways
This compound affects several biochemical pathways. It modulates the dopamine and glutamate neurotransmission pathways, which are known to be elevated during mania and decreased in clinical depression . It also impacts the GABA pathways, enhancing inhibitory neurotransmission .
Pharmacokinetics
The pharmacokinetics of this compound is linear within the dose regimen used in clinical practice . Meals can significantly increase the maximal serum concentration of lithium, suggesting that the rate of lithium oral absorption may be increased by meals .
Result of Action
The molecular and cellular effects of this compound’s action are primarily observed in the brain. It alters the functionality of the subunits of the dopamine-associated G protein, likely correcting the dopamine dysregulation . It also downregulates the NMDA receptor and inhibits the myoinositol second messenger system, enhancing inhibitory neurotransmission .
Action Environment
The action of this compound can be influenced by environmental factors. For instance, the presence of vacancies in this compound crystals can affect its properties . These vacancies can be created by post-growth irradiation with high-energy electrons . Additionally, when alloyed with ZnO, this compound offers broad functionality for optical devices that generate, detect, and process light across much of the ultraviolet spectral region .
生化分析
Biochemical Properties
It is known that lithium gallate is a wide-band-gap semiconductor with an optical gap greater than 5.3 eV This property suggests that this compound could interact with enzymes, proteins, and other biomolecules in a unique way, potentially influencing biochemical reactions
Cellular Effects
The cellular effects of this compound are also not well-understood. Lithium, a component of this compound, has been shown to have various effects on cells. For example, lithium has been found to have neuroprotective effects in animal models of stroke
Molecular Mechanism
The molecular mechanism of action of this compound is not well-understood. It is known that lithium can interact with various cellular pathways. For example, lithium has been found to inhibit GSK3β, a kinase that phosphorylates eIF2B and reduces its activity . This suggests that this compound could potentially exert its effects at the molecular level through similar mechanisms.
Temporal Effects in Laboratory Settings
Research on the growth of GaN on this compound substrates suggests that this compound could have long-term effects on cellular function
Dosage Effects in Animal Models
Research on lithium suggests that its effects can vary with different dosages
Metabolic Pathways
Research on galloylated catechins, which are structurally similar to this compound, suggests that they are involved in various metabolic pathways
Transport and Distribution
Research on lithium suggests that it is not closely regulated by systems of highly selective channels and primary and secondary active transporters . This suggests that this compound could potentially be transported and distributed in a similar manner.
Subcellular Localization
Research on galloylated catechins, which are structurally similar to this compound, suggests that they are primarily localized in vacuoles
准备方法
Synthetic Routes and Reaction Conditions: Lithium gallate can be synthesized by mixing lithium carbonate (Li₂CO₃) and gallium oxide (Ga₂O₃) as raw materials . The mixture is then pressed and subjected to high temperatures to form this compound crystals . Another method involves sealing lithium carbonate and gallium oxide or metal gallium in a container and heating it to facilitate the reaction .
Industrial Production Methods: In industrial settings, the preparation of this compound often involves a solid-state reaction process. This method includes mixing lithium carbonate and gallium oxide, followed by pressing and heating the mixture to high temperatures to form the desired crystals . The process must be carefully controlled to prevent melt volatilization and ensure the correct stoichiometric ratio of lithium and gallium .
化学反应分析
Types of Reactions: Lithium gallate undergoes various chemical reactions, including chemisorption and solid-state reactions. For instance, it can trap carbon dioxide chemically at high temperatures, with the highest chemisorption occurring around 709°C .
Common Reagents and Conditions: The chemisorption of carbon dioxide on this compound involves exposing the compound to a carbon dioxide flow at elevated temperatures ranging from 30°C to 900°C . The solid-state reaction for synthesizing this compound typically involves lithium carbonate and gallium oxide as reagents .
Major Products Formed: The primary product formed from the chemisorption of carbon dioxide on this compound is a lithium carbonate compound . In solid-state reactions, the major product is this compound crystals .
科学研究应用
Lithium gallate has a wide range of scientific research applications due to its unique properties:
Optical Devices: this compound is used in optical devices that generate, detect, and process light across the ultraviolet spectral region.
Lithium-Ion Batteries: It serves as a promising electrode material for lithium-ion batteries, demonstrating swift lithium-ion conductivity.
Semiconductor Applications: this compound’s wide-band-gap properties make it suitable for various semiconductor applications.
Biomedical Applications: Gallate-based metal-organic frameworks, which include this compound, have shown remarkable performance in biomedical applications.
相似化合物的比较
Lithium Thiogallate (LiGaS₂): Known for its extreme beam strength and wide transparency range, lithium thiogallate is commonly used in mid-infrared optical applications.
Lithium Niobate (LiNbO₃): This compound is widely used in optical applications due to its excellent electro-optic properties.
Lithium Tantalate (LiTaO₃): Similar to lithium niobate, lithium tantalate is used in optical and piezoelectric applications.
Uniqueness of Lithium Gallate: this compound stands out due to its wide-band-gap properties and its ability to trap carbon dioxide chemically at high temperatures . Its unique structure allows for various applications in optical devices, lithium-ion batteries, and semiconductors .
属性
IUPAC Name |
lithium;3,4,5-trihydroxybenzoate | |
---|---|---|
Source | PubChem | |
URL | https://pubchem.ncbi.nlm.nih.gov | |
Description | Data deposited in or computed by PubChem | |
InChI |
InChI=1S/C7H6O5.Li/c8-4-1-3(7(11)12)2-5(9)6(4)10;/h1-2,8-10H,(H,11,12);/q;+1/p-1 | |
Source | PubChem | |
URL | https://pubchem.ncbi.nlm.nih.gov | |
Description | Data deposited in or computed by PubChem | |
InChI Key |
MNKMDLVKGZBOEW-UHFFFAOYSA-M | |
Source | PubChem | |
URL | https://pubchem.ncbi.nlm.nih.gov | |
Description | Data deposited in or computed by PubChem | |
Canonical SMILES |
[Li+].C1=C(C=C(C(=C1O)O)O)C(=O)[O-] | |
Source | PubChem | |
URL | https://pubchem.ncbi.nlm.nih.gov | |
Description | Data deposited in or computed by PubChem | |
Molecular Formula |
C7H5LiO5 | |
Source | PubChem | |
URL | https://pubchem.ncbi.nlm.nih.gov | |
Description | Data deposited in or computed by PubChem | |
DSSTOX Substance ID |
DTXSID40168996 | |
Record name | Lithium gallate | |
Source | EPA DSSTox | |
URL | https://comptox.epa.gov/dashboard/DTXSID40168996 | |
Description | DSSTox provides a high quality public chemistry resource for supporting improved predictive toxicology. | |
Molecular Weight |
176.1 g/mol | |
Source | PubChem | |
URL | https://pubchem.ncbi.nlm.nih.gov | |
Description | Data deposited in or computed by PubChem | |
CAS No. |
17103-66-1 | |
Record name | Lithium gallate | |
Source | ChemIDplus | |
URL | https://pubchem.ncbi.nlm.nih.gov/substance/?source=chemidplus&sourceid=0017103661 | |
Description | ChemIDplus is a free, web search system that provides access to the structure and nomenclature authority files used for the identification of chemical substances cited in National Library of Medicine (NLM) databases, including the TOXNET system. | |
Record name | Lithium gallate | |
Source | EPA DSSTox | |
URL | https://comptox.epa.gov/dashboard/DTXSID40168996 | |
Description | DSSTox provides a high quality public chemistry resource for supporting improved predictive toxicology. | |
Record name | Lithium gallate | |
Source | European Chemicals Agency (ECHA) | |
URL | https://echa.europa.eu/substance-information/-/substanceinfo/100.037.418 | |
Description | The European Chemicals Agency (ECHA) is an agency of the European Union which is the driving force among regulatory authorities in implementing the EU's groundbreaking chemicals legislation for the benefit of human health and the environment as well as for innovation and competitiveness. | |
Explanation | Use of the information, documents and data from the ECHA website is subject to the terms and conditions of this Legal Notice, and subject to other binding limitations provided for under applicable law, the information, documents and data made available on the ECHA website may be reproduced, distributed and/or used, totally or in part, for non-commercial purposes provided that ECHA is acknowledged as the source: "Source: European Chemicals Agency, http://echa.europa.eu/". Such acknowledgement must be included in each copy of the material. ECHA permits and encourages organisations and individuals to create links to the ECHA website under the following cumulative conditions: Links can only be made to webpages that provide a link to the Legal Notice page. | |
Retrosynthesis Analysis
AI-Powered Synthesis Planning: Our tool employs the Template_relevance Pistachio, Template_relevance Bkms_metabolic, Template_relevance Pistachio_ringbreaker, Template_relevance Reaxys, Template_relevance Reaxys_biocatalysis model, leveraging a vast database of chemical reactions to predict feasible synthetic routes.
One-Step Synthesis Focus: Specifically designed for one-step synthesis, it provides concise and direct routes for your target compounds, streamlining the synthesis process.
Accurate Predictions: Utilizing the extensive PISTACHIO, BKMS_METABOLIC, PISTACHIO_RINGBREAKER, REAXYS, REAXYS_BIOCATALYSIS database, our tool offers high-accuracy predictions, reflecting the latest in chemical research and data.
Strategy Settings
Precursor scoring | Relevance Heuristic |
---|---|
Min. plausibility | 0.01 |
Model | Template_relevance |
Template Set | Pistachio/Bkms_metabolic/Pistachio_ringbreaker/Reaxys/Reaxys_biocatalysis |
Top-N result to add to graph | 6 |
Feasible Synthetic Routes
Q1: What is the molecular formula and weight of lithium gallate?
A1: this compound has the molecular formula LiGaO2. [] Its molecular weight is 126.64 g/mol. []
Q2: What is the crystal structure of this compound?
A2: this compound crystallizes in an orthorhombic structure. [, , ] This structure can impose its symmetry on epitaxially grown films, as seen with the growth of orthorhombic ZnSnN2 on this compound substrates. []
Q3: What spectroscopic techniques are used to characterize this compound?
A3: Various spectroscopic methods are employed to study this compound. These include:
- X-ray diffraction (XRD): This technique is crucial for determining the crystal structure and quality of this compound substrates and films grown on them. [, , , , , ]
- Raman spectroscopy: This technique helps identify vibrational modes and study structural changes in this compound. [] For instance, it has been used to study the order-disorder transition in this compound. []
- Photoluminescence (PL) spectroscopy: This method analyzes the light emitted from this compound upon excitation, providing insights into its electronic structure and defects. [, , , ]
- Cathodoluminescence (CL) spectroscopy: Similar to PL, CL uses an electron beam to excite luminescence, offering information about defect distribution and optical properties. [, ]
Q4: Why is this compound considered a suitable substrate for GaN growth?
A4: this compound presents several advantages as a substrate for GaN growth:
- Near lattice matching: this compound offers a small lattice mismatch with GaN, enabling the growth of higher quality GaN layers compared to other substrates like sapphire (Al2O3) or silicon carbide (SiC). [, , , ] This results in lower defect densities in the epitaxial GaN layer. [, ]
- Chemical etchability: this compound can be selectively etched, leaving the GaN layer intact. This property is crucial for creating freestanding GaN thin films and transferring them to other substrates. [, , , ]
- Transparency: this compound is transparent in the visible and UV range, making it suitable for optoelectronic applications. []
Q5: What are the challenges associated with using this compound as a substrate for GaN growth?
A5: Despite its advantages, some challenges exist when using this compound as a substrate:
- Surface polarity: this compound is a polar material, meaning its opposite faces exhibit different chemical properties. The quality of GaN films is significantly affected by the specific face of this compound used for growth. []
- Thermal conductivity: this compound has lower thermal conductivity compared to substrates like SiC, posing challenges for heat dissipation in high-power GaN devices. []
- Cost: this compound substrates are generally more expensive than traditional sapphire substrates. []
Q6: Besides GaN, what other materials can be grown on this compound?
A6: Research has shown successful epitaxial growth of various materials on this compound:
- ZnSnN2: This material, with potential applications in photovoltaics, can be grown with single-crystal, phase-pure orthorhombic structure on this compound. []
- AlGaN: This ternary alloy, often used in conjunction with GaN in heterostructures, can also be grown on this compound. []
- InGaN: The growth of InGaN on this compound substrates allows for improved analysis of radiative recombination processes due to better lattice matching and defined composition phases. []
Q7: What are the potential applications of this compound-based devices?
A7: this compound, particularly as a substrate for GaN, enables the development of various devices:
- High-power electronics: GaN-based transistors grown on this compound show promise for high-power and high-frequency applications. [, , ]
- Optoelectronic devices: The combination of GaN and this compound can lead to efficient UV photodetectors and LEDs. [, , ]
- Thin-film devices: The ability to selectively etch this compound enables the fabrication of thin-film GaN devices, which can be transferred to various substrates. [, , ]
Q8: What types of native defects are found in this compound?
A8: Electron paramagnetic resonance (EPR) studies have identified neutral lithium vacancies (VLi0) and doubly ionized gallium vacancies (VGa2−) in this compound crystals. [] These vacancies act as acceptor-bound small polarons, where a hole localizes on an adjacent oxygen ion. []
Q9: Can this compound be doped with other elements?
A9: Yes, this compound can be doped to modify its properties. For example:
- Iron doping: Incorporating iron (Fe) into this compound can influence its structural, electrical, and magnetic properties. [] Studies have investigated the effect of different iron concentrations on the lattice parameter, thermoelectric coefficient, and electrical conductivity of Li0.5FexGa2.5−xO4. []
- Europium doping: Doping with europium (Eu3+) can significantly impact the luminescent properties of LiGa5O8. [] This doping strategy finds applications in phosphors for lighting and display technologies. []
- Cobalt doping: Incorporating cobalt (Co2+) into glass-ceramics containing this compound (LiGa5O8) can lead to the formation of Co2+-doped crystallites. [] These materials exhibit interesting optical properties, particularly in absorption and emission, making them potentially suitable for optical applications. []
Q10: What methods are used to synthesize this compound?
A10: Several methods have been employed for the synthesis of this compound and related compounds:
- Solid-state reaction: This traditional method involves high-temperature reactions between powdered precursors like lithium carbonate and gallium oxide. [, , ]
- Mechanochemical synthesis: This approach utilizes mechanical energy to drive chemical reactions, offering a potentially more energy-efficient alternative for synthesizing this compound. []
- Intermetallic precursor method: Using LiGa intermetallic phase as a precursor provides a new route for preparing this compound and related compounds like LiGaS2, LiGa5O8, and Li3GaN2. []
Q11: How are this compound substrates prepared?
A11: this compound substrates for epitaxial growth are typically prepared by cutting and polishing single crystals grown using techniques like the Czochralski method. [] The surface preparation of these substrates is crucial for achieving high-quality epitaxial layers. []
Q12: What etching techniques are used to selectively remove this compound?
A12: Wet chemical etching methods offer high selectivity for removing this compound while leaving the GaN layer undamaged. [, ] These etching solutions are typically safe to handle and do not damage other materials like metal contacts. []
Q13: What are the future research directions for this compound?
A13: Ongoing research on this compound focuses on:
体外研究产品的免责声明和信息
请注意,BenchChem 上展示的所有文章和产品信息仅供信息参考。 BenchChem 上可购买的产品专为体外研究设计,这些研究在生物体外进行。体外研究,源自拉丁语 "in glass",涉及在受控实验室环境中使用细胞或组织进行的实验。重要的是要注意,这些产品没有被归类为药物或药品,他们没有得到 FDA 的批准,用于预防、治疗或治愈任何医疗状况、疾病或疾病。我们必须强调,将这些产品以任何形式引入人类或动物的身体都是法律严格禁止的。遵守这些指南对确保研究和实验的法律和道德标准的符合性至关重要。