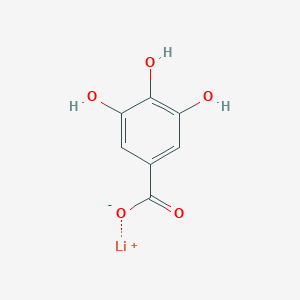
Lithium gallate
Descripción general
Descripción
Lithium gallate (LGO) is a compound that has garnered interest due to its compatibility with gallium nitride (GaN) and potential applications in electronics. It serves as a substrate for GaN growth and can be used in thin-film production through substrate removal or lift-off processes . The compound is part of the lithium-gallium binary system, which includes various stoichiometric phases with significant ranges of stoichiometry . This compound does not form electron-precise Zintl phases, and its electronic structure combines ionic, covalent, and metallic bonding contributions .
Synthesis Analysis
The synthesis of this compound-related compounds involves various methods, including electrochemical techniques and reactions with organometallic compounds. For instance, the electrochemical insertion of lithium into gallium has been studied, showing a preference for the lighter lithium isotope during the process . Additionally, the reaction between tert-butylphosphonic acid and LiGaMe4 in organic solvents yields a molecular lithium gallium phosphonate, which is a potential precursor for molecular sieves and ion conductors . Other synthesis methods include the reaction of lithium organoaluminates and gallium compounds to form base-free lithium-organoaluminate and gallium congeners .
Molecular Structure Analysis
The molecular structure of this compound-related compounds has been extensively studied using X-ray diffraction and other analytical techniques. For example, the molecular structure of a lithium gallium phosphonate cage has been determined, revealing a one-dimensional wire of coordinated lithium ions . Similarly, the crystal structures of various Ga-rich lithium gallides have been elucidated, showing new structure types and confirming their air- and moisture-sensitive nature .
Chemical Reactions Analysis
This compound and its related compounds exhibit interesting reactivity. For instance, this compound substrates can be selectively etched using wet etching techniques, which is crucial for the production of GaN thin films . The reactivity of lithium gallium phosphonate compounds towards air, moisture, and other reagents has also been investigated, showing stability under certain conditions and transformation under others .
Physical and Chemical Properties Analysis
The physical and chemical properties of this compound and related compounds are influenced by their synthesis and molecular structure. The electrochemical investigation of the lithium-gallium system has provided insights into the thermodynamic properties, including phase behavior and mass transport parameters . The addition of gallium to lithium-stuffed garnets has been shown to stabilize the cubic garnet-type phase and improve lithium ion conductivity . Furthermore, the specific electronic resistivities of lithium gallides have been measured, confirming their metallic behavior .
Aplicaciones Científicas De Investigación
High Frequency, High Power Electronic Applications Lithium gallate is gaining attention for III-nitride material growth due to its superior lattice match. This makes it a promising candidate for power electronic applications, as it allows for the production of high-quality, extremely thin nitride materials (Doolittle et al., 2000).
Semiconductor Properties LiGaO₂ is a wide-band-gap semiconductor with significant potential in optical devices. Its optical gap is greater than 5.3 eV, and when alloyed with ZnO, it can be used in devices that generate, detect, and process light across much of the ultraviolet spectral region (Lenyk et al., 2018).
Optical and Display Technologies The addition of lithium to zinc gallate thin-film phosphors has shown to enhance photo- and low-voltage cathodoluminescent intensity, making it suitable for applications in low-voltage field emission display devices (Lee et al., 2002).
Fabrication of Optical Waveguides Gallium oxide, when used as a diffusion source for the fabrication of lithium niobate optical waveguides, can lead to the development of single-mode single-polarization optical waveguides with low propagation loss (Huang & Wang, 2007).
Chemical Reactions and Properties LiGaO₂ also plays a role in various chemical reactions and properties, such as in the synthesis of iodonium tetrakis(pentafluorophenyl)gallates, which are photoinitiators with potential applications in various fields (Ren et al., 2002).
Safety and Hazards
Direcciones Futuras
Lithium-ion battery cell formation is one of the most critical process steps in lithium-ion battery (LIB) cell production . It affects the key battery performance metrics, e.g. rate capability, lifetime and safety, is time-consuming and contributes significantly to energy consumption during cell production and overall cell cost . As LIBs usually exceed the electrochemical stability window of the electrolyte, formation is required to activate and stabilize the electrochemical reactions .
Mecanismo De Acción
Target of Action
Lithium Gallate, also known as lithium;3,4,5-trihydroxybenzoate, primarily targets neurotransmitters and second messenger systems in the brain . It interacts with dopamine and glutamate pathways, which play crucial roles in the pathogenesis of bipolar affective disorder . This compound also affects GABA pathways, an inhibitory transmitter that modulates glutamate and dopamine .
Mode of Action
This compound exerts its effects by competing for macromolecular sites that are relatively specific for other cations, especially sodium and magnesium . In the dopamine pathway, it decreases presynaptic dopamine activity and inactivates postsynaptic G protein, reducing excitatory neurotransmission in the brain . In the glutamate pathway, it enhances inhibitory neurotransmission by downregulating the NMDA receptor and inhibiting the myoinositol second messenger system directly .
Biochemical Pathways
This compound affects several biochemical pathways. It modulates the dopamine and glutamate neurotransmission pathways, which are known to be elevated during mania and decreased in clinical depression . It also impacts the GABA pathways, enhancing inhibitory neurotransmission .
Pharmacokinetics
The pharmacokinetics of this compound is linear within the dose regimen used in clinical practice . Meals can significantly increase the maximal serum concentration of lithium, suggesting that the rate of lithium oral absorption may be increased by meals .
Result of Action
The molecular and cellular effects of this compound’s action are primarily observed in the brain. It alters the functionality of the subunits of the dopamine-associated G protein, likely correcting the dopamine dysregulation . It also downregulates the NMDA receptor and inhibits the myoinositol second messenger system, enhancing inhibitory neurotransmission .
Action Environment
The action of this compound can be influenced by environmental factors. For instance, the presence of vacancies in this compound crystals can affect its properties . These vacancies can be created by post-growth irradiation with high-energy electrons . Additionally, when alloyed with ZnO, this compound offers broad functionality for optical devices that generate, detect, and process light across much of the ultraviolet spectral region .
Propiedades
IUPAC Name |
lithium;3,4,5-trihydroxybenzoate | |
---|---|---|
Source | PubChem | |
URL | https://pubchem.ncbi.nlm.nih.gov | |
Description | Data deposited in or computed by PubChem | |
InChI |
InChI=1S/C7H6O5.Li/c8-4-1-3(7(11)12)2-5(9)6(4)10;/h1-2,8-10H,(H,11,12);/q;+1/p-1 | |
Source | PubChem | |
URL | https://pubchem.ncbi.nlm.nih.gov | |
Description | Data deposited in or computed by PubChem | |
InChI Key |
MNKMDLVKGZBOEW-UHFFFAOYSA-M | |
Source | PubChem | |
URL | https://pubchem.ncbi.nlm.nih.gov | |
Description | Data deposited in or computed by PubChem | |
Canonical SMILES |
[Li+].C1=C(C=C(C(=C1O)O)O)C(=O)[O-] | |
Source | PubChem | |
URL | https://pubchem.ncbi.nlm.nih.gov | |
Description | Data deposited in or computed by PubChem | |
Molecular Formula |
C7H5LiO5 | |
Source | PubChem | |
URL | https://pubchem.ncbi.nlm.nih.gov | |
Description | Data deposited in or computed by PubChem | |
DSSTOX Substance ID |
DTXSID40168996 | |
Record name | Lithium gallate | |
Source | EPA DSSTox | |
URL | https://comptox.epa.gov/dashboard/DTXSID40168996 | |
Description | DSSTox provides a high quality public chemistry resource for supporting improved predictive toxicology. | |
Molecular Weight |
176.1 g/mol | |
Source | PubChem | |
URL | https://pubchem.ncbi.nlm.nih.gov | |
Description | Data deposited in or computed by PubChem | |
CAS RN |
17103-66-1 | |
Record name | Lithium gallate | |
Source | ChemIDplus | |
URL | https://pubchem.ncbi.nlm.nih.gov/substance/?source=chemidplus&sourceid=0017103661 | |
Description | ChemIDplus is a free, web search system that provides access to the structure and nomenclature authority files used for the identification of chemical substances cited in National Library of Medicine (NLM) databases, including the TOXNET system. | |
Record name | Lithium gallate | |
Source | EPA DSSTox | |
URL | https://comptox.epa.gov/dashboard/DTXSID40168996 | |
Description | DSSTox provides a high quality public chemistry resource for supporting improved predictive toxicology. | |
Record name | Lithium gallate | |
Source | European Chemicals Agency (ECHA) | |
URL | https://echa.europa.eu/substance-information/-/substanceinfo/100.037.418 | |
Description | The European Chemicals Agency (ECHA) is an agency of the European Union which is the driving force among regulatory authorities in implementing the EU's groundbreaking chemicals legislation for the benefit of human health and the environment as well as for innovation and competitiveness. | |
Explanation | Use of the information, documents and data from the ECHA website is subject to the terms and conditions of this Legal Notice, and subject to other binding limitations provided for under applicable law, the information, documents and data made available on the ECHA website may be reproduced, distributed and/or used, totally or in part, for non-commercial purposes provided that ECHA is acknowledged as the source: "Source: European Chemicals Agency, http://echa.europa.eu/". Such acknowledgement must be included in each copy of the material. ECHA permits and encourages organisations and individuals to create links to the ECHA website under the following cumulative conditions: Links can only be made to webpages that provide a link to the Legal Notice page. | |
Retrosynthesis Analysis
AI-Powered Synthesis Planning: Our tool employs the Template_relevance Pistachio, Template_relevance Bkms_metabolic, Template_relevance Pistachio_ringbreaker, Template_relevance Reaxys, Template_relevance Reaxys_biocatalysis model, leveraging a vast database of chemical reactions to predict feasible synthetic routes.
One-Step Synthesis Focus: Specifically designed for one-step synthesis, it provides concise and direct routes for your target compounds, streamlining the synthesis process.
Accurate Predictions: Utilizing the extensive PISTACHIO, BKMS_METABOLIC, PISTACHIO_RINGBREAKER, REAXYS, REAXYS_BIOCATALYSIS database, our tool offers high-accuracy predictions, reflecting the latest in chemical research and data.
Strategy Settings
Precursor scoring | Relevance Heuristic |
---|---|
Min. plausibility | 0.01 |
Model | Template_relevance |
Template Set | Pistachio/Bkms_metabolic/Pistachio_ringbreaker/Reaxys/Reaxys_biocatalysis |
Top-N result to add to graph | 6 |
Feasible Synthetic Routes
Q & A
Q1: What is the molecular formula and weight of lithium gallate?
A1: this compound has the molecular formula LiGaO2. [] Its molecular weight is 126.64 g/mol. []
Q2: What is the crystal structure of this compound?
A2: this compound crystallizes in an orthorhombic structure. [, , ] This structure can impose its symmetry on epitaxially grown films, as seen with the growth of orthorhombic ZnSnN2 on this compound substrates. []
Q3: What spectroscopic techniques are used to characterize this compound?
A3: Various spectroscopic methods are employed to study this compound. These include:
- X-ray diffraction (XRD): This technique is crucial for determining the crystal structure and quality of this compound substrates and films grown on them. [, , , , , ]
- Raman spectroscopy: This technique helps identify vibrational modes and study structural changes in this compound. [] For instance, it has been used to study the order-disorder transition in this compound. []
- Photoluminescence (PL) spectroscopy: This method analyzes the light emitted from this compound upon excitation, providing insights into its electronic structure and defects. [, , , ]
- Cathodoluminescence (CL) spectroscopy: Similar to PL, CL uses an electron beam to excite luminescence, offering information about defect distribution and optical properties. [, ]
Q4: Why is this compound considered a suitable substrate for GaN growth?
A4: this compound presents several advantages as a substrate for GaN growth:
- Near lattice matching: this compound offers a small lattice mismatch with GaN, enabling the growth of higher quality GaN layers compared to other substrates like sapphire (Al2O3) or silicon carbide (SiC). [, , , ] This results in lower defect densities in the epitaxial GaN layer. [, ]
- Chemical etchability: this compound can be selectively etched, leaving the GaN layer intact. This property is crucial for creating freestanding GaN thin films and transferring them to other substrates. [, , , ]
- Transparency: this compound is transparent in the visible and UV range, making it suitable for optoelectronic applications. []
Q5: What are the challenges associated with using this compound as a substrate for GaN growth?
A5: Despite its advantages, some challenges exist when using this compound as a substrate:
- Surface polarity: this compound is a polar material, meaning its opposite faces exhibit different chemical properties. The quality of GaN films is significantly affected by the specific face of this compound used for growth. []
- Thermal conductivity: this compound has lower thermal conductivity compared to substrates like SiC, posing challenges for heat dissipation in high-power GaN devices. []
- Cost: this compound substrates are generally more expensive than traditional sapphire substrates. []
Q6: Besides GaN, what other materials can be grown on this compound?
A6: Research has shown successful epitaxial growth of various materials on this compound:
- ZnSnN2: This material, with potential applications in photovoltaics, can be grown with single-crystal, phase-pure orthorhombic structure on this compound. []
- AlGaN: This ternary alloy, often used in conjunction with GaN in heterostructures, can also be grown on this compound. []
- InGaN: The growth of InGaN on this compound substrates allows for improved analysis of radiative recombination processes due to better lattice matching and defined composition phases. []
Q7: What are the potential applications of this compound-based devices?
A7: this compound, particularly as a substrate for GaN, enables the development of various devices:
- High-power electronics: GaN-based transistors grown on this compound show promise for high-power and high-frequency applications. [, , ]
- Optoelectronic devices: The combination of GaN and this compound can lead to efficient UV photodetectors and LEDs. [, , ]
- Thin-film devices: The ability to selectively etch this compound enables the fabrication of thin-film GaN devices, which can be transferred to various substrates. [, , ]
Q8: What types of native defects are found in this compound?
A8: Electron paramagnetic resonance (EPR) studies have identified neutral lithium vacancies (VLi0) and doubly ionized gallium vacancies (VGa2−) in this compound crystals. [] These vacancies act as acceptor-bound small polarons, where a hole localizes on an adjacent oxygen ion. []
Q9: Can this compound be doped with other elements?
A9: Yes, this compound can be doped to modify its properties. For example:
- Iron doping: Incorporating iron (Fe) into this compound can influence its structural, electrical, and magnetic properties. [] Studies have investigated the effect of different iron concentrations on the lattice parameter, thermoelectric coefficient, and electrical conductivity of Li0.5FexGa2.5−xO4. []
- Europium doping: Doping with europium (Eu3+) can significantly impact the luminescent properties of LiGa5O8. [] This doping strategy finds applications in phosphors for lighting and display technologies. []
- Cobalt doping: Incorporating cobalt (Co2+) into glass-ceramics containing this compound (LiGa5O8) can lead to the formation of Co2+-doped crystallites. [] These materials exhibit interesting optical properties, particularly in absorption and emission, making them potentially suitable for optical applications. []
Q10: What methods are used to synthesize this compound?
A10: Several methods have been employed for the synthesis of this compound and related compounds:
- Solid-state reaction: This traditional method involves high-temperature reactions between powdered precursors like lithium carbonate and gallium oxide. [, , ]
- Mechanochemical synthesis: This approach utilizes mechanical energy to drive chemical reactions, offering a potentially more energy-efficient alternative for synthesizing this compound. []
- Intermetallic precursor method: Using LiGa intermetallic phase as a precursor provides a new route for preparing this compound and related compounds like LiGaS2, LiGa5O8, and Li3GaN2. []
Q11: How are this compound substrates prepared?
A11: this compound substrates for epitaxial growth are typically prepared by cutting and polishing single crystals grown using techniques like the Czochralski method. [] The surface preparation of these substrates is crucial for achieving high-quality epitaxial layers. []
Q12: What etching techniques are used to selectively remove this compound?
A12: Wet chemical etching methods offer high selectivity for removing this compound while leaving the GaN layer undamaged. [, ] These etching solutions are typically safe to handle and do not damage other materials like metal contacts. []
Q13: What are the future research directions for this compound?
A13: Ongoing research on this compound focuses on:
Descargo de responsabilidad e información sobre productos de investigación in vitro
Tenga en cuenta que todos los artículos e información de productos presentados en BenchChem están destinados únicamente con fines informativos. Los productos disponibles para la compra en BenchChem están diseñados específicamente para estudios in vitro, que se realizan fuera de organismos vivos. Los estudios in vitro, derivados del término latino "in vidrio", involucran experimentos realizados en entornos de laboratorio controlados utilizando células o tejidos. Es importante tener en cuenta que estos productos no se clasifican como medicamentos y no han recibido la aprobación de la FDA para la prevención, tratamiento o cura de ninguna condición médica, dolencia o enfermedad. Debemos enfatizar que cualquier forma de introducción corporal de estos productos en humanos o animales está estrictamente prohibida por ley. Es esencial adherirse a estas pautas para garantizar el cumplimiento de los estándares legales y éticos en la investigación y experimentación.