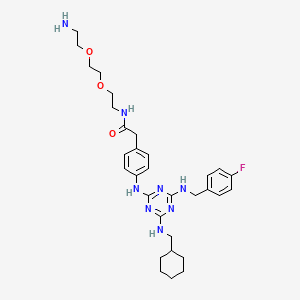
AP-III-a4
- Click on QUICK INQUIRY to receive a quote from our team of experts.
- With the quality product at a COMPETITIVE price, you can focus more on your research.
Overview
Description
AP-III-a4, also known as ENOblock, is a small molecule inhibitor of the enzyme enolase. It is the first nonsubstrate analogue inhibitor of enolase with an IC50 value of 0.576 micromolar. Enolase is a crucial enzyme in the glycolytic pathway, and its inhibition has significant implications for cellular metabolism and cancer treatment .
Mechanism of Action
Target of Action
AP-III-a4 primarily targets the enzyme Enolase 1 (ENO1) and Enolase 2 (ENO2), which are crucial enzymes in the process of glycolysis . ENO1 and ENO2 have been implicated in cancer progression, and their dysregulation has been reported in multiple cancers .
Mode of Action
this compound directly binds to ENO1 and ENO2, inhibiting their catalytic activity . This inhibition results in a decrease in cancer cell aggressiveness, as ENO1 and ENO2 are essential for cancer cell survival . Furthermore, this compound induces cell death under hypoxia and inhibits cancer cell migration and invasion by down-regulating AKT and Bcl-xL expression .
Biochemical Pathways
this compound affects the glycolytic pathway by inhibiting the activity of ENO1 and ENO2 . This inhibition leads to a blockade in PKM2-mediated glycolytic flux and CCND1-associated cell cycle progression . In addition, this compound can induce glucose uptake and inhibit phosphoenolpyruvate carboxykinase (PEPCK) expression in hepatocytes and kidney cells .
Pharmacokinetics
It is known that this compound can be used for the research of cancer and diabetes
Result of Action
The inhibition of ENO1 and ENO2 by this compound results in a reduction in cancer cell aggressiveness and an increase in cell death . It also inhibits cancer cell migration and invasion . Furthermore, this compound treatment can reduce the stemness of cancer cells, which is a significant biomarker associated with the stemness of cancer cells .
Action Environment
The action of this compound is influenced by the tumor microenvironment. For instance, this compound induces higher levels of cell death in hypoxic conditions compared to normoxia . The expression level of ENO1, a target of this compound, is correlated with the infiltration levels of immune cells and immune-related functions . This suggests that the tumor immune microenvironment can influence the action, efficacy, and stability of this compound .
Biochemical Analysis
Biochemical Properties
AP-III-a4 directly binds to enolase and inhibits its activity . It has an IC50 value of 0.576 μM . In HCT116 cells, this compound has been shown to induce cell death under hypoxic conditions and inhibit cancer cell migration and invasion by downregulating AKT and Bcl-xL expression .
Cellular Effects
This compound has been shown to induce glucose uptake and inhibit phosphoenolpyruvate carboxykinase (PEPCK) expression in hepatocytes and kidney cells . In HCT116 cells, it induces cell death under hypoxic conditions and inhibits cancer cell migration and invasion .
Molecular Mechanism
The molecular mechanism of this compound involves direct binding to enolase and inhibition of its activity . This leads to a decrease in the expression of AKT and Bcl-xL, which are negative regulators of apoptosis .
Temporal Effects in Laboratory Settings
The effects of this compound over time in laboratory settings have not been extensively studied. It has been shown to induce cell death in HCT116 cells under hypoxic conditions within 24 hours of treatment .
Dosage Effects in Animal Models
In a HCT116-xenotransplanted zebrafish tumor xenograft model, this compound at a dosage of 10 μM was shown to inhibit cancer cell migration and invasion .
Metabolic Pathways
This compound is involved in the glycolytic pathway, where it inhibits the activity of enolase . This leads to a decrease in the expression of PEPCK, a key enzyme in gluconeogenesis .
Preparation Methods
Synthetic Routes and Reaction Conditions
The synthesis of AP-III-a4 involves multiple steps, starting from commercially available starting materials. The key steps include the formation of the core structure through a series of condensation and cyclization reactions, followed by functional group modifications to achieve the final product. The reaction conditions typically involve the use of organic solvents, catalysts, and controlled temperatures to ensure high yield and purity .
Industrial Production Methods
Industrial production of this compound follows similar synthetic routes but on a larger scale. The process is optimized for efficiency and cost-effectiveness, with stringent quality control measures to ensure consistency and purity. The use of automated reactors and continuous flow systems can enhance the scalability of the production process .
Chemical Reactions Analysis
Types of Reactions
AP-III-a4 undergoes various chemical reactions, including:
Oxidation: this compound can be oxidized under specific conditions to form oxidized derivatives.
Reduction: Reduction reactions can convert this compound to its reduced forms.
Substitution: this compound can participate in substitution reactions where functional groups are replaced with other groups.
Common Reagents and Conditions
Oxidation: Common oxidizing agents include hydrogen peroxide and potassium permanganate.
Reduction: Reducing agents such as sodium borohydride and lithium aluminum hydride are used.
Substitution: Various nucleophiles and electrophiles can be used for substitution reactions, depending on the desired product.
Major Products Formed
The major products formed from these reactions depend on the specific reagents and conditions used. For example, oxidation may yield hydroxylated or ketone derivatives, while reduction may produce alcohols or amines .
Scientific Research Applications
AP-III-a4 has a wide range of scientific research applications, including:
Cancer Research: this compound inhibits enolase, which is involved in cancer cell metabolism.
Diabetes Research: This compound has been studied for its potential to regulate glucose metabolism and improve insulin sensitivity, making it a promising candidate for diabetes treatment.
Neurodegenerative Diseases: Enolase is also implicated in neurodegenerative diseases, and this compound’s inhibition of enolase may have therapeutic potential in conditions such as Alzheimer’s disease.
Metabolic Disorders: This compound’s ability to modulate glycolysis and other metabolic pathways makes it a valuable tool for studying metabolic disorders.
Comparison with Similar Compounds
Similar Compounds
POMHEX: Another enolase inhibitor with anticancer activity.
Hex: A compound that binds to enolase and inhibits its activity.
D-(-)-3-Phosphoglyceric acid disodium: An enolase inhibitor used in metabolic studies.
Rosmarinic acid: A natural compound with enolase inhibitory activity.
Uniqueness of AP-III-a4
This compound is unique in its ability to inhibit enolase without being a substrate analogue. This property allows it to effectively disrupt the glycolytic pathway and exert its anticancer and metabolic effects. Its high specificity and potency make it a valuable tool for research and potential therapeutic applications .
Properties
IUPAC Name |
N-[2-[2-(2-aminoethoxy)ethoxy]ethyl]-2-[4-[[4-(cyclohexylmethylamino)-6-[(4-fluorophenyl)methylamino]-1,3,5-triazin-2-yl]amino]phenyl]acetamide |
Source
|
---|---|---|
Source | PubChem | |
URL | https://pubchem.ncbi.nlm.nih.gov | |
Description | Data deposited in or computed by PubChem | |
InChI |
InChI=1S/C31H43FN8O3/c32-26-10-6-25(7-11-26)22-36-30-38-29(35-21-24-4-2-1-3-5-24)39-31(40-30)37-27-12-8-23(9-13-27)20-28(41)34-15-17-43-19-18-42-16-14-33/h6-13,24H,1-5,14-22,33H2,(H,34,41)(H3,35,36,37,38,39,40) |
Source
|
Source | PubChem | |
URL | https://pubchem.ncbi.nlm.nih.gov | |
Description | Data deposited in or computed by PubChem | |
InChI Key |
MOVYITHKOHMLHC-UHFFFAOYSA-N |
Source
|
Source | PubChem | |
URL | https://pubchem.ncbi.nlm.nih.gov | |
Description | Data deposited in or computed by PubChem | |
Canonical SMILES |
C1CCC(CC1)CNC2=NC(=NC(=N2)NCC3=CC=C(C=C3)F)NC4=CC=C(C=C4)CC(=O)NCCOCCOCCN |
Source
|
Source | PubChem | |
URL | https://pubchem.ncbi.nlm.nih.gov | |
Description | Data deposited in or computed by PubChem | |
Molecular Formula |
C31H43FN8O3 |
Source
|
Source | PubChem | |
URL | https://pubchem.ncbi.nlm.nih.gov | |
Description | Data deposited in or computed by PubChem | |
Molecular Weight |
594.7 g/mol |
Source
|
Source | PubChem | |
URL | https://pubchem.ncbi.nlm.nih.gov | |
Description | Data deposited in or computed by PubChem | |
Q1: What is AP-III-a4 and how does it interact with ENO1?
A1: this compound is a small molecule inhibitor of α-enolase (ENO1) enzymatic activity. While its precise mechanism of action requires further investigation, it likely binds to the active site of ENO1, hindering its ability to catalyze the conversion of 2-phosphoglycerate to phosphoenolpyruvate in the glycolytic pathway. This inhibition was shown to have downstream effects on the PI3K/AKT pathway, a crucial signaling cascade involved in cell growth and survival. []
Q2: The research mentions that B7-H3 regulates ENO1 activity. How does inhibiting ENO1 with this compound impact the effects of B7-H3 in lung cancer cells?
A2: The study by [] demonstrated that B7-H3, a protein often overexpressed in lung cancer, can promote cancer cell proliferation and migration partly by enhancing ENO1 activity. When researchers applied this compound to block ENO1 activity, the effects of B7-H3 on both PI3K/AKT pathway activation and lung cancer cell proliferation and migration were significantly diminished. This suggests that ENO1 activity is a key mediator of B7-H3's oncogenic effects in this context.
Disclaimer and Information on In-Vitro Research Products
Please be aware that all articles and product information presented on BenchChem are intended solely for informational purposes. The products available for purchase on BenchChem are specifically designed for in-vitro studies, which are conducted outside of living organisms. In-vitro studies, derived from the Latin term "in glass," involve experiments performed in controlled laboratory settings using cells or tissues. It is important to note that these products are not categorized as medicines or drugs, and they have not received approval from the FDA for the prevention, treatment, or cure of any medical condition, ailment, or disease. We must emphasize that any form of bodily introduction of these products into humans or animals is strictly prohibited by law. It is essential to adhere to these guidelines to ensure compliance with legal and ethical standards in research and experimentation.