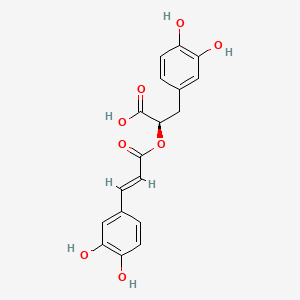
Rosmarinic acid
Overview
Description
Rosmarinic acid (RA), a polyphenolic ester of caffeic acid and 3,4-dihydroxyphenyllactic acid, is a widely studied natural compound renowned for its antioxidant, anti-inflammatory, and neuroprotective properties . It is abundant in plants of the Lamiaceae family, such as rosemary (Rosmarinus officinalis), sage (Salvia officinalis), and basil (Ocimum basilicum). Its mechanisms include scavenging reactive oxygen species (ROS), modulating inflammatory cytokines (e.g., TNF-α, IL-6), and inhibiting enzymes like α-glucosidase and caspase-3 .
Preparation Methods
Synthetic Routes and Reaction Conditions: Rosmarinic acid can be synthesized through an esterification reaction between caffeic acid and 3,4-dihydroxyphenyl lactic acid. The reaction typically involves the use of a catalyst, such as sulfuric acid, and is carried out under reflux conditions . The synthetic route can be summarized as follows:
- Esterification of caffeic acid with 3,4-dihydroxyphenyl lactic acid.
- Purification of the resulting this compound through recrystallization.
Industrial Production Methods: Industrial production of this compound often involves the extraction from plant sources. The extraction process includes:
- Harvesting and drying the plant material.
- Grinding the dried material to a fine powder.
- Extracting the this compound using solvents such as ethanol or acetone.
- Purifying the extract through filtration and evaporation .
Chemical Reactions Analysis
Radical Scavenging Mechanisms
RA’s antioxidant activity is mediated through three primary pathways: Hydrogen Atom Transfer (HAT) , Single Electron Transfer (SET) , and Proton Loss Electron Transfer (PLET) .
Hydrogen Atom Transfer (HAT)
RA neutralizes free radicals (e.g., HO- , HOO- , CH3OO- ) by donating hydrogen atoms from its hydroxyl groups. Key findings include:
-
Bond Dissociation Energies (BDE) :
-
Rate Constants :
Radical Type | Reaction Pathway | Rate Constant (M⁻¹s⁻¹) |
---|---|---|
HO- | HAT | 2.89 × 10¹⁰ |
HOO- | HAT/SET | 3.18 × 10⁸ |
CH3OO- | HAT | 3.16 × 10⁴ (lipid) |
Single Electron Transfer (SET)
RA’s dianion form facilitates SET mechanisms, particularly in aqueous environments. Computational studies indicate:
-
Reduction Potential : RA exhibits lower reduction potentials than ascorbate, enabling efficient electron transfer to neutralize radicals .
-
Marcus Theory Parameters :
Metal Chelation and Redox Modulation
RA chelates transition metals (Fe²⁺, Fe³⁺, Cu²⁺), inhibiting Fenton/Haber-Weiss reactions that generate HO- radicals. Key reactions include:
Iron Complexation
RA binds Fe³⁺ via ester and phenolic hydroxyl groups, forming stable complexes with:
-
Formation Constant (Kf) : High values reflect strong chelation.
-
Thermodynamic Parameters :
Copper Chelation
RA interacts with Cu²⁺, disrupting Fenton-like reactions. NMR studies show broadening of RA’s aromatic protons upon Cu²⁺ addition, confirming binding .
Metal Ion | Chelation Site | Effect on ROS Generation |
---|---|---|
Fe³⁺ | Phenolic OH groups | Inhibits Fenton reaction |
Cu²⁺ | Ester and phenolic | Reduces HO- production |
Pro-Oxidant Risks
RA’s redox dualism depends on environmental conditions:
-
Fe(III)-to-Fe(II) Reduction : RA reduces Fe³⁺ to Fe²⁺ in the presence of superoxide (O₂⁻), initiating Fenton-like HO- generation .
-
Ascorbate Interaction : RA inhibits Cu²⁺ reduction by ascorbate, mitigating pro-oxidant risks via the OIL-1 pathway .
Reducing Agent | Pro-Oxidant Risk |
---|---|
Superoxide (O₂⁻) | High (Fe³⁺ reduction) |
Ascorbate (AA⁻) | Low (Cu²⁺ inhibition) |
Structural Interactions
RA binds biomolecules like bovine serum albumin (BSA) with high affinity (Kf = 4.18 × 10⁴ M⁻¹), interacting at subdomain IIA residues . This suggests potential applications in drug delivery and protein protection.
Scientific Research Applications
Antioxidant Properties
Rosmarinic acid is renowned for its potent antioxidant capabilities. It helps scavenge free radicals and protects cells from oxidative damage. This property is crucial in mitigating the effects of chronic diseases associated with oxidative stress.
- Mechanism : RA directly counteracts reactive oxygen species (ROS) and enhances cellular antioxidant defenses, thereby reducing oxidative damage in non-tumor tissues while augmenting the efficacy of chemotherapy in tumor tissues .
Anti-inflammatory Effects
RA exhibits significant anti-inflammatory properties by inhibiting pro-inflammatory cytokines and enzymes such as lipoxygenase and cyclooxygenases. This makes it a valuable compound in managing inflammatory diseases.
- Applications : RA's anti-inflammatory effects have been studied in conditions like arthritis and inflammatory bowel disease, highlighting its potential as a therapeutic agent .
Antimicrobial Activity
This compound demonstrates broad-spectrum antimicrobial activity against various pathogens, including bacteria, viruses, and fungi.
- Case Study : A study highlighted that RA effectively inhibited the growth of Staphylococcus aureus and Escherichia coli at minimal inhibitory concentrations (MIC) ranging from 0.1 to 1.0 mg/mL .
Pathogen | MIC (mg/mL) | Reference |
---|---|---|
Staphylococcus aureus | 1.0 | |
Escherichia coli | 0.8 | |
Candida albicans | 0.1 | |
SARS-CoV-2 | 25.47 ng/μL |
Anticancer Applications
Research indicates that this compound can play a significant role in cancer treatment by enhancing the efficacy of chemotherapeutic agents and reducing resistance to these drugs.
- Mechanism : RA regulates oxidative stress, chronic inflammation, cell cycle progression, apoptosis, and metastasis in cancer cells. It has been shown to reverse chemoresistance mechanisms in various cancer types .
Neuroprotective Effects
This compound has been investigated for its neuroprotective properties, particularly in the context of neurodegenerative diseases such as Alzheimer's disease.
Mechanism of Action
Rosmarinic acid is often compared with other polyphenolic compounds such as:
Caffeic Acid: Both compounds share similar antioxidant properties, but this compound has a more complex structure, providing additional biological activities.
Chlorogenic Acid: Similar in antioxidant activity, but this compound has superior anti-inflammatory and antimicrobial properties.
Ferulic Acid: Both have antioxidant properties, but this compound is more effective in inhibiting lipid peroxidation
Comparison with Similar Compounds
Structural Similarities and Differences
RA shares structural motifs with several phenolic compounds, particularly catechol-containing molecules. Key comparisons include:
Compound | Core Structure | Key Functional Groups | Molecular Weight |
---|---|---|---|
Rosmarinic acid | Caffeic acid esterified with 3,4-dihydroxyphenyllactic acid | Catechol, carboxylic acid | 360.3 g/mol |
Carnosic acid | Abietane diterpene | Catechol, diterpene skeleton | 332.4 g/mol |
Salvianolic acid B | Tanshinone derivative | Multiple phenolic hydroxyls | 718.6 g/mol |
Quercetin | Flavonol | Catechol (B-ring), ketone | 302.2 g/mol |
RA and carnosic acid both feature catechol groups, enabling hydrogen donation to neutralize free radicals . Salvianolic acid B, a structurally complex polyphenol, shares RA’s antioxidant properties but differs in its triterpenoid backbone .
Pharmacological Activity Comparison
2.2.1. Antioxidant and Anti-inflammatory Effects
- RA vs. Carnosic Acid: Both compounds inhibit nitric oxide (NO) production in macrophages and microglia, but RA exhibits stronger direct ROS scavenging, while carnosic acid shows superior lipophilic antioxidant activity due to its diterpene structure .
- RA vs. Quercetin : RA’s esterified structure enhances bioavailability compared to quercetin, which undergoes rapid glucuronidation .
2.2.2. Neuroprotection
- RA vs. Carnosic Acid: RA inhibits caspase-3 activation (IC₅₀ = 2.1 µM) more effectively than carnosic acid, making it a potent candidate for Alzheimer’s disease therapy . Both compounds mitigate nitrosative stress but differ in cellular targets: RA primarily acts on neurons, whereas carnosic acid modulates microglial activation .
2.2.3. Enzyme Inhibition
- α-Amylase Inhibition: RA in oregano extracts inhibits porcine pancreatic amylase (PPA) 40% more effectively than purified RA, suggesting synergism with other phenolics (e.g., carvacrol) .
- α-Glucosidase Inhibition : RA (IC₅₀ = 0.8 mM) outperforms chlorogenic acid (IC₅₀ = 1.2 mM) in delaying carbohydrate digestion .
Content Variability in Plant Sources
RA content varies significantly across species and extraction conditions:
Ethanol concentration critically impacts RA yield: concentrations >70% reduce RA solubility, while hydro-ethanol mixtures (30–50% ethanol) optimize extraction .
Synergistic Interactions
- RA and carnosic acid in rosemary extracts synergistically enhance antioxidant and anti-inflammatory effects, reducing required doses by 50% in murine models .
- RA combined with quercetin in O. aristatus amplifies α-glucosidase inhibition, achieving 85% activity reduction compared to 60% for RA alone .
Key Research Findings
Neurodegenerative Diseases: RA reduces β-amyloid plaques in Alzheimer’s models by 40% via caspase-3 inhibition, outperforming carnosic acid (25% reduction) .
Diabetes Management : RA’s dual inhibition of α-amylase and α-glucosidase (IC₅₀ = 0.8–1.2 mM) positions it as a multitarget antidiabetic agent .
Cancer Therapy: RA induces apoptosis in colorectal cancer cells (IC₅₀ = 50 µM) through ROS-mediated pathways, with efficacy comparable to salvianolic acid B .
Biological Activity
Rosmarinic acid (RA) is a polyphenolic compound found in various plants, particularly in the mint family, and is known for its diverse biological activities. This article explores the biological activity of this compound, focusing on its antioxidant, anti-inflammatory, anticancer, antimicrobial, and neuroprotective properties, supported by recent research findings and case studies.
1. Antioxidant Activity
This compound exhibits significant antioxidant properties, which are crucial for protecting cells from oxidative stress. Studies have demonstrated that RA can reduce the production of reactive oxygen species (ROS) and enhance the activity of antioxidant enzymes.
- Mechanism of Action: RA scavenges free radicals due to its O-diphenol hydroxyl groups, effectively entering lipid bilayers to exert its antioxidant effects .
- Research Findings: In a study involving human dopaminergic SH-SY5Y cells, RA was shown to mitigate oxidative stress induced by hydrogen peroxide (H2O2) by decreasing ROS levels and regulating apoptotic proteins such as Bax and Bcl-2 .
2. Anti-inflammatory Effects
RA has been extensively studied for its anti-inflammatory properties. It has been shown to inhibit pro-inflammatory pathways and cytokine production.
- Inhibition of COX-2: Research indicates that RA can inhibit cyclo-oxygenase-2 (COX-2) activity, which is often elevated in inflammatory conditions .
- Case Study: A study highlighted the effectiveness of RA in reducing inflammation markers in models of vascular dementia, suggesting its potential therapeutic role in neurodegenerative diseases .
3. Anticancer Potential
The anticancer activity of this compound has gained attention due to its ability to inhibit tumor growth and induce apoptosis in various cancer cell lines.
- Breast Cancer: RA significantly inhibited the proliferation of MDA-MB-231 breast cancer cells in a dose-dependent manner and induced apoptosis by modulating Bcl-2 and caspase pathways .
- Liver Cancer: In human liver cancer Huh7 cells, RA was found to promote apoptosis and inhibit cell proliferation through similar apoptotic mechanisms .
Cancer Type | Effect of this compound | Mechanism |
---|---|---|
Breast Cancer | Inhibition of MDA-MB-231 cell proliferation | Induction of apoptosis |
Liver Cancer | Inhibition of Huh7 cell growth | Regulation of Bcl-2/Caspase pathway |
Prostate Cancer | Inhibition of PC3 cell activity | Modulation of MAPK pathway |
4. Antimicrobial Activity
RA demonstrates significant antimicrobial properties against a variety of pathogens.
- Bacterial Inhibition: Studies have shown that RA possesses antibacterial effects against common pathogens such as Staphylococcus aureus and Escherichia coli .
- Antiviral Properties: RA has also been noted for its antiviral activity, particularly against viruses like herpes simplex virus (HSV) and human immunodeficiency virus (HIV) .
5. Neuroprotective Effects
The neuroprotective potential of this compound is particularly noteworthy in the context of neurodegenerative diseases.
- Mechanism: RA protects neurons from oxidative stress-induced damage by modulating signaling pathways involved in neuronal survival .
- Research Findings: In models of amyloid-beta-induced neurotoxicity, RA was effective in reducing cytotoxicity and preserving neuronal function .
6. Summary of Biological Activities
This compound's multifaceted biological activities make it a compound of interest for therapeutic applications. Below is a summary table highlighting its key activities:
Q & A
Basic Research Questions
Q. What are the standard analytical methods for quantifying rosmarinic acid in plant extracts, and how are they validated?
High-performance liquid chromatography (HPLC) with photodiode array detection is the most widely used method for this compound quantification. Key validation parameters include linearity (R² > 0.99), precision (%RSD < 2%), and recovery rates (90–110%). Gradient elution is common for complex matrices, while isocratic methods are optimized for speed and reproducibility in specific plant families like Lamiaceae . Comparative studies with LC/MS/MS are recommended for cross-validation, particularly when analyzing low-concentration samples .
Q. Which biological activities of this compound are most supported by in vitro evidence?
Robust in vitro evidence exists for its antioxidant (via DPPH/ABTS radical scavenging), antimicrobial (membrane permeability disruption), and anti-inflammatory (NF-κB pathway inhibition) properties. For example, this compound increases bacterial membrane permeability, leading to carbohydrate/protein leakage (validated via spectrophotometric assays at 260/280 nm) . Anti-inflammatory effects are quantified through TNF-α and IL-6 suppression in macrophage models .
Q. What are the key considerations when designing in vivo studies on this compound’s neuroprotective effects?
Use murine models (e.g., LPS-induced neuroinflammation) with endpoints like blood-brain barrier permeability (Evans Blue assay) and cytokine levels (ELISA). Dose optimization (10–50 mg/kg body weight) and bioavailability enhancement (liposomal encapsulation) are critical due to this compound’s moderate log P (1.76) and rapid metabolism .
Advanced Research Questions
Q. How can researchers resolve contradictions in reported antioxidant capacities of this compound across studies?
Discrepancies often arise from assay-specific reactivity (e.g., ORAC vs. FRAP) and solvent polarity effects. Standardize protocols using the same radical source (e.g., DPPH in methanol) and control for pH/temperature. Quantum chemical calculations (DFT) can identify dominant antioxidant mechanisms (HAT vs. SET) by analyzing spin density distributions on phenolic hydroxyl groups .
Q. What molecular docking approaches validate this compound’s interaction with viral replication proteins?
Use PyMOL or AutoDock Vina to simulate binding with target proteins (e.g., Hepatitis E’s Tyrosine FYN). Validate docking poses via RMSD (<2 Å) and binding energy (<−7 kcal/mol). Hydrogen bonding with residues like ASN-154 and hydrophobic interactions with LEU-120 are critical. Complement with in vitro SPR assays to measure affinity (KD < 1 µM) .
Q. How does this compound influence bacterial protein metabolism?
Proteomic analysis (2D gel electrophoresis and LC-MS/MS) reveals degradation of ribosomal proteins (e.g., 30S subunit) and upregulation of stress-response chaperones (GroEL). Dose-dependent effects are observed at MIC values of 0.5–2 mg/mL, with SDS-PAGE showing truncated protein bands after 24-hour exposure .
Q. What spectroscopic techniques beyond HPLC are validated for this compound quantification?
Near-infrared (NIR) and attenuated total reflection infrared (ATR-IR) spectroscopy coupled with partial least squares (PLS) regression achieve R² > 0.90 in plant matrices. Key NIR wavelengths include 1,650 nm (C=O stretching) and 2,100 nm (aromatic C-H). ATR-IR peaks at 1,610 cm⁻¹ (phenolic ring vibrations) are diagnostic. Cross-validate with HPLC to ensure accuracy (SEP < 0.06%) .
Q. What computational chemistry methods elucidate this compound’s antioxidant mechanism?
Density functional theory (DFT) at the B3LYP/6-311++G(d,p) level calculates bond dissociation enthalpy (BDE) and ionization potential (IP) for phenolic OH groups. Spin density maps identify active sites (C4-OH in the caffeoyl moiety). Molecular dynamics simulations in lipid bilayers assess membrane permeability, correlating with experimental antioxidant kinetics in oil-in-water emulsions .
Q. Methodological Notes
- Data Contradiction Analysis : Compare studies using the same model (e.g., RAW 264.7 macrophages for anti-inflammatory assays) and normalize data to positive controls (e.g., ascorbic acid for antioxidant studies) .
- Experimental Reproducibility : Document extraction solvents (e.g., 70% ethanol vs. methanol) and lyophilization protocols, as these significantly impact this compound yield (10–72 mg/g variation across Lamiaceae species) .
Properties
IUPAC Name |
(2R)-3-(3,4-dihydroxyphenyl)-2-[(E)-3-(3,4-dihydroxyphenyl)prop-2-enoyl]oxypropanoic acid | |
---|---|---|
Source | PubChem | |
URL | https://pubchem.ncbi.nlm.nih.gov | |
Description | Data deposited in or computed by PubChem | |
InChI |
InChI=1S/C18H16O8/c19-12-4-1-10(7-14(12)21)3-6-17(23)26-16(18(24)25)9-11-2-5-13(20)15(22)8-11/h1-8,16,19-22H,9H2,(H,24,25)/b6-3+/t16-/m1/s1 | |
Source | PubChem | |
URL | https://pubchem.ncbi.nlm.nih.gov | |
Description | Data deposited in or computed by PubChem | |
InChI Key |
DOUMFZQKYFQNTF-WUTVXBCWSA-N | |
Source | PubChem | |
URL | https://pubchem.ncbi.nlm.nih.gov | |
Description | Data deposited in or computed by PubChem | |
Canonical SMILES |
C1=CC(=C(C=C1CC(C(=O)O)OC(=O)C=CC2=CC(=C(C=C2)O)O)O)O | |
Source | PubChem | |
URL | https://pubchem.ncbi.nlm.nih.gov | |
Description | Data deposited in or computed by PubChem | |
Isomeric SMILES |
C1=CC(=C(C=C1C[C@H](C(=O)O)OC(=O)/C=C/C2=CC(=C(C=C2)O)O)O)O | |
Source | PubChem | |
URL | https://pubchem.ncbi.nlm.nih.gov | |
Description | Data deposited in or computed by PubChem | |
Molecular Formula |
C18H16O8 | |
Source | PubChem | |
URL | https://pubchem.ncbi.nlm.nih.gov | |
Description | Data deposited in or computed by PubChem | |
DSSTOX Substance ID |
DTXSID20896987 | |
Record name | Rosmarinic acid | |
Source | EPA DSSTox | |
URL | https://comptox.epa.gov/dashboard/DTXSID20896987 | |
Description | DSSTox provides a high quality public chemistry resource for supporting improved predictive toxicology. | |
Molecular Weight |
360.3 g/mol | |
Source | PubChem | |
URL | https://pubchem.ncbi.nlm.nih.gov | |
Description | Data deposited in or computed by PubChem | |
Physical Description |
Solid | |
Record name | Rosmarinic acid | |
Source | Human Metabolome Database (HMDB) | |
URL | http://www.hmdb.ca/metabolites/HMDB0003572 | |
Description | The Human Metabolome Database (HMDB) is a freely available electronic database containing detailed information about small molecule metabolites found in the human body. | |
Explanation | HMDB is offered to the public as a freely available resource. Use and re-distribution of the data, in whole or in part, for commercial purposes requires explicit permission of the authors and explicit acknowledgment of the source material (HMDB) and the original publication (see the HMDB citing page). We ask that users who download significant portions of the database cite the HMDB paper in any resulting publications. | |
Solubility |
Soluble in ethanol, DMSO or dimethyl formamide to approximately 25 mg/mL | |
Record name | ROSMARINIC ACID | |
Source | Hazardous Substances Data Bank (HSDB) | |
URL | https://pubchem.ncbi.nlm.nih.gov/source/hsdb/7688 | |
Description | The Hazardous Substances Data Bank (HSDB) is a toxicology database that focuses on the toxicology of potentially hazardous chemicals. It provides information on human exposure, industrial hygiene, emergency handling procedures, environmental fate, regulatory requirements, nanomaterials, and related areas. The information in HSDB has been assessed by a Scientific Review Panel. | |
Density |
1.547 g/cu cm | |
Record name | ROSMARINIC ACID | |
Source | Hazardous Substances Data Bank (HSDB) | |
URL | https://pubchem.ncbi.nlm.nih.gov/source/hsdb/7688 | |
Description | The Hazardous Substances Data Bank (HSDB) is a toxicology database that focuses on the toxicology of potentially hazardous chemicals. It provides information on human exposure, industrial hygiene, emergency handling procedures, environmental fate, regulatory requirements, nanomaterials, and related areas. The information in HSDB has been assessed by a Scientific Review Panel. | |
Mechanism of Action |
... To determine the effects of rosmarinic acid on melanogenesis and elucidate the molecular events of melanogenesis induced by rosmarinic acid, several experiments were performed in B16 melanoma cells. In this study, ... the melanin content and tyrosinase expression were increased by rosmarinic acid in a concentration-dependent manner. In addition, after the melanin content was increased by rosmarinic acid, it was reduced by H-89 and KT 5720, protein kinase A (PKA) inhibitors, but not by SB203580, a p38mapk inhibitor, or Ro-32-0432, a PKC inhibitor, which suggests the involvement of PKA in rosmarinic acid-induced melanogenesis. Consistent with this, rosmarinic acid induced the phosphorylation of CRE-binding protein (CREB), but had no effect on the phosphorylation of p38mapk or the inhibition of Akt phosphorylation. Additionally, rosmarinic acid induced the activation of cAMP response element (CRE) without having any effect on cAMP production, which suggests that rosmarinic acid-induced melanogenesis is mediated by PKA, which occurs downstream of cAMP production. This result was further confirmed by the fact that rosmarinic acid-induced phosphorylation of CREB was inhibited by H-89, but not by PD98059, a MEK1 inhibitor, or by LY294002, a phosphatidylinositol-3-kinase (PI3K) inhibitor. Rosmarinic acid-induced expression of tyrosinase protein was attenuated by H-89. Based on these results, ... rosmarinic acid induces melanogenesis through PKA activation signaling., Rosmarinic acid (RA) ... inhibits several complement-dependent inflammatory processes and may have potential as a therapeutic agent for the control of complement activation in disease. Rosmarinic acid has been reported to have effects on both the classical pathway C3-convertase and on the cobra venom factor-induced, alternative pathway convertase. In order to define the mechanism of inhibition, the effect of RA on classical and alternative pathway lysis, C1q binding, the classical pathway convertase, the alternative pathway convertase, membrane attack pathway lysis and the generation of fragments of C3 and C5 during activation, was tested in vitro. The results showed that RA inhibited lysis by the classical pathway more than by the alternative pathway. This effect was dose-dependent with maximum inhibition of classical pathway lysis observed at 2.6 mmoles of RA. There was little effect on C1q binding or on the classical and alternative pathway convertases. However, there was highly significant inhibition of lysis of pre-formed EA43b cells by dilutions of human or rabbit serum in the presence of RA (1 mM); this was accompanied by inhibition of C5a generation. /It was concluded/ that the inhibitory effect of RA involves the C5 convertase. Such inhibition could be advantageous to the host in disorders where the terminal attack sequence plays a role in pathogenesis., ...Rosmarinic acid (RA), a naturally occurring polyphenol flavonoid, has been reported to inhibit TNF-alpha-induced NF-kappaB activation in human dermal fibroblasts. However, the precise mechanisms of RA have not been well elucidated in TNF-alpha-mediated anti-cancer therapy. In this study, /the authors/ found that RA treatment significantly sensitizes TNF-alpha-induced apoptosis in human leukemia U937 cells through the suppression of nuclear transcription factor-kappaB (NF-kappaB) and reactive oxygen species (ROS). Activation of caspases in response to TNF-alpha was markedly increased by RA treatment. However, pretreatment with the caspase-3 inhibitor, z-DEVD-fmk, was capable of significantly restoring cell viability in response to combined treatment. RA also suppressed NF-kappaB activation through inhibition of phosphorylation and degradation of IkappaBalpha, and nuclear translocation of p50 and p65. This inhibition was correlated with suppression of NF-kappaB-dependent anti-apoptotic proteins (IAP-1, IAP-2, and XIAP). RA treatment also normalized TNF-alpha-induced ROS generation. Additionally, ectopic Bcl-2 expressing U937 reversed combined treatment-induced cell death, cytochrome c release into cytosol, and collapse of mitochondrial potential. These results demonstrated that RA inhibits TNF-alpha-induced ROS generation and NF-kappaB activation, and enhances TNF-alpha-induced apoptosis., Rosmarinic acid (RosA) is a hydroxylated compound frequently found in herbal plants and is mostly responsible for anti-inflammatory and antioxidative activity. Previously... RosA inhibited T-cell antigen receptor (TCR)- induced interleukin 2 (IL-2) expression and subsequent T-cell proliferation in vitro. /This study/ investigated /the/ inhibitory mechanism of RosA on TCR signaling, which ultimately activates IL-2 promoter by activating transcription factors, such as nuclear factor of activated T cells (NF-AT) and activating protein-1 (AP-1). Interestingly, RosA inhibited NF-AT activation but not AP-1, suggesting that RosA inhibits Ca+2-dependent signaling pathways only. Signaling events upstream of NF-AT activation, such as the generation of inositol 1,4,5-triphosphate and Ca+2 mobilization, and tyrosine phosphorylation of phospholipase C-gamma 1 (PLC-gamma 1) were strongly inhibited by RosA. Tyrosine phosphorylation of PLC-gamma 1 is largely dependent on 3 kinds of protein tyrosine kinases (PTKs), ie, Lck, ZAP-70, and Itk. /Investigators/ found that RosA efficiently inhibited TCR-induced tyrosine phosphorylation and subsequent activation of Itk but did not inhibit Lck or ZAP-70. ZAP-70-dependent signaling pathways such as the tyrosine phosphorylation of LAT and SLP-76 and serine/threonine phosphorylation of mitogen-activated protein kinases (MAPKs) were intact in the presence of RosA, confirming that RosA suppresses TCR signaling in a ZAP-70-independent manner. .../It is concluded/ that RosA inhibits TCR signaling leading to Ca+2 mobilization and NF-AT activation by blocking membrane-proximal events, specifically, the tyrosine phosphorylation of inducible T cells kinase (Itk) and PLC-gamma 1. | |
Record name | ROSMARINIC ACID | |
Source | Hazardous Substances Data Bank (HSDB) | |
URL | https://pubchem.ncbi.nlm.nih.gov/source/hsdb/7688 | |
Description | The Hazardous Substances Data Bank (HSDB) is a toxicology database that focuses on the toxicology of potentially hazardous chemicals. It provides information on human exposure, industrial hygiene, emergency handling procedures, environmental fate, regulatory requirements, nanomaterials, and related areas. The information in HSDB has been assessed by a Scientific Review Panel. | |
Color/Form |
Crystalline solid | |
CAS No. |
20283-92-5, 537-15-5 | |
Record name | Rosmarinic acid | |
Source | CAS Common Chemistry | |
URL | https://commonchemistry.cas.org/detail?cas_rn=20283-92-5 | |
Description | CAS Common Chemistry is an open community resource for accessing chemical information. Nearly 500,000 chemical substances from CAS REGISTRY cover areas of community interest, including common and frequently regulated chemicals, and those relevant to high school and undergraduate chemistry classes. This chemical information, curated by our expert scientists, is provided in alignment with our mission as a division of the American Chemical Society. | |
Explanation | The data from CAS Common Chemistry is provided under a CC-BY-NC 4.0 license, unless otherwise stated. | |
Record name | Rosmarinic acid | |
Source | ChemIDplus | |
URL | https://pubchem.ncbi.nlm.nih.gov/substance/?source=chemidplus&sourceid=0000537155 | |
Description | ChemIDplus is a free, web search system that provides access to the structure and nomenclature authority files used for the identification of chemical substances cited in National Library of Medicine (NLM) databases, including the TOXNET system. | |
Record name | Rosmarinic acid | |
Source | ChemIDplus | |
URL | https://pubchem.ncbi.nlm.nih.gov/substance/?source=chemidplus&sourceid=0020283925 | |
Description | ChemIDplus is a free, web search system that provides access to the structure and nomenclature authority files used for the identification of chemical substances cited in National Library of Medicine (NLM) databases, including the TOXNET system. | |
Record name | Rosmarinic acid | |
Source | DrugBank | |
URL | https://www.drugbank.ca/drugs/DB16865 | |
Description | The DrugBank database is a unique bioinformatics and cheminformatics resource that combines detailed drug (i.e. chemical, pharmacological and pharmaceutical) data with comprehensive drug target (i.e. sequence, structure, and pathway) information. | |
Explanation | Creative Common's Attribution-NonCommercial 4.0 International License (http://creativecommons.org/licenses/by-nc/4.0/legalcode) | |
Record name | Rosmarinic acid | |
Source | EPA DSSTox | |
URL | https://comptox.epa.gov/dashboard/DTXSID20896987 | |
Description | DSSTox provides a high quality public chemistry resource for supporting improved predictive toxicology. | |
Record name | Benzenepropanoic acid, α-[[(2E)-3-(3,4-dihydroxyphenyl)-1-oxo-2-propen-1-yl]oxy]-3,4-dihydroxy-, (αR) | |
Source | European Chemicals Agency (ECHA) | |
URL | https://echa.europa.eu/substance-information/-/substanceinfo/100.123.507 | |
Description | The European Chemicals Agency (ECHA) is an agency of the European Union which is the driving force among regulatory authorities in implementing the EU's groundbreaking chemicals legislation for the benefit of human health and the environment as well as for innovation and competitiveness. | |
Explanation | Use of the information, documents and data from the ECHA website is subject to the terms and conditions of this Legal Notice, and subject to other binding limitations provided for under applicable law, the information, documents and data made available on the ECHA website may be reproduced, distributed and/or used, totally or in part, for non-commercial purposes provided that ECHA is acknowledged as the source: "Source: European Chemicals Agency, http://echa.europa.eu/". Such acknowledgement must be included in each copy of the material. ECHA permits and encourages organisations and individuals to create links to the ECHA website under the following cumulative conditions: Links can only be made to webpages that provide a link to the Legal Notice page. | |
Record name | ROSMARINIC ACID | |
Source | FDA Global Substance Registration System (GSRS) | |
URL | https://gsrs.ncats.nih.gov/ginas/app/beta/substances/MQE6XG29YI | |
Description | The FDA Global Substance Registration System (GSRS) enables the efficient and accurate exchange of information on what substances are in regulated products. Instead of relying on names, which vary across regulatory domains, countries, and regions, the GSRS knowledge base makes it possible for substances to be defined by standardized, scientific descriptions. | |
Explanation | Unless otherwise noted, the contents of the FDA website (www.fda.gov), both text and graphics, are not copyrighted. They are in the public domain and may be republished, reprinted and otherwise used freely by anyone without the need to obtain permission from FDA. Credit to the U.S. Food and Drug Administration as the source is appreciated but not required. | |
Record name | ROSMARINIC ACID | |
Source | Hazardous Substances Data Bank (HSDB) | |
URL | https://pubchem.ncbi.nlm.nih.gov/source/hsdb/7688 | |
Description | The Hazardous Substances Data Bank (HSDB) is a toxicology database that focuses on the toxicology of potentially hazardous chemicals. It provides information on human exposure, industrial hygiene, emergency handling procedures, environmental fate, regulatory requirements, nanomaterials, and related areas. The information in HSDB has been assessed by a Scientific Review Panel. | |
Record name | Rosmarinic acid | |
Source | Human Metabolome Database (HMDB) | |
URL | http://www.hmdb.ca/metabolites/HMDB0003572 | |
Description | The Human Metabolome Database (HMDB) is a freely available electronic database containing detailed information about small molecule metabolites found in the human body. | |
Explanation | HMDB is offered to the public as a freely available resource. Use and re-distribution of the data, in whole or in part, for commercial purposes requires explicit permission of the authors and explicit acknowledgment of the source material (HMDB) and the original publication (see the HMDB citing page). We ask that users who download significant portions of the database cite the HMDB paper in any resulting publications. | |
Melting Point |
171-175 °C | |
Record name | ROSMARINIC ACID | |
Source | Hazardous Substances Data Bank (HSDB) | |
URL | https://pubchem.ncbi.nlm.nih.gov/source/hsdb/7688 | |
Description | The Hazardous Substances Data Bank (HSDB) is a toxicology database that focuses on the toxicology of potentially hazardous chemicals. It provides information on human exposure, industrial hygiene, emergency handling procedures, environmental fate, regulatory requirements, nanomaterials, and related areas. The information in HSDB has been assessed by a Scientific Review Panel. | |
Retrosynthesis Analysis
AI-Powered Synthesis Planning: Our tool employs the Template_relevance Pistachio, Template_relevance Bkms_metabolic, Template_relevance Pistachio_ringbreaker, Template_relevance Reaxys, Template_relevance Reaxys_biocatalysis model, leveraging a vast database of chemical reactions to predict feasible synthetic routes.
One-Step Synthesis Focus: Specifically designed for one-step synthesis, it provides concise and direct routes for your target compounds, streamlining the synthesis process.
Accurate Predictions: Utilizing the extensive PISTACHIO, BKMS_METABOLIC, PISTACHIO_RINGBREAKER, REAXYS, REAXYS_BIOCATALYSIS database, our tool offers high-accuracy predictions, reflecting the latest in chemical research and data.
Strategy Settings
Precursor scoring | Relevance Heuristic |
---|---|
Min. plausibility | 0.01 |
Model | Template_relevance |
Template Set | Pistachio/Bkms_metabolic/Pistachio_ringbreaker/Reaxys/Reaxys_biocatalysis |
Top-N result to add to graph | 6 |
Feasible Synthetic Routes
Disclaimer and Information on In-Vitro Research Products
Please be aware that all articles and product information presented on BenchChem are intended solely for informational purposes. The products available for purchase on BenchChem are specifically designed for in-vitro studies, which are conducted outside of living organisms. In-vitro studies, derived from the Latin term "in glass," involve experiments performed in controlled laboratory settings using cells or tissues. It is important to note that these products are not categorized as medicines or drugs, and they have not received approval from the FDA for the prevention, treatment, or cure of any medical condition, ailment, or disease. We must emphasize that any form of bodily introduction of these products into humans or animals is strictly prohibited by law. It is essential to adhere to these guidelines to ensure compliance with legal and ethical standards in research and experimentation.