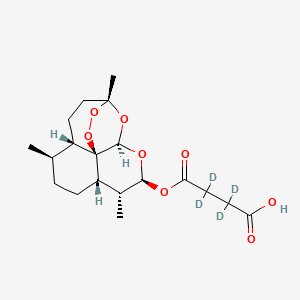
Artesunate-d4
Overview
Description
Artesunate-d4 is a deuterium-labeled derivative of artesunate, a semisynthetic artemisinin compound. Artesunate is widely known for its potent antimalarial properties and is used in the treatment of severe malaria. The deuterium labeling in this compound makes it particularly useful in analytical chemistry for tracing and quantification purposes .
Preparation Methods
Synthetic Routes and Reaction Conditions
Artesunate-d4 is synthesized through a series of chemical reactions starting from dihydroartemisinin, which is derived from artemisinin, the active component of the Chinese medicinal herb Artemisia annua. The synthesis involves the reduction of artemisinin to dihydroartemisinin, followed by esterification with succinic anhydride to form artesunate. The deuterium labeling is introduced during the synthesis process to replace specific hydrogen atoms with deuterium .
Industrial Production Methods
Industrial production of this compound follows similar synthetic routes as described above but on a larger scale. The process involves stringent quality control measures to ensure the purity and consistency of the final product. The use of deuterium-labeled compounds in industrial applications is primarily for analytical method development, method validation, and quality control .
Chemical Reactions Analysis
Types of Reactions
Artesunate-d4 undergoes various chemical reactions, including:
Oxidation: this compound can be oxidized to form dihydroartemisinin derivatives.
Reduction: Reduction reactions can convert this compound back to its precursor compounds.
Substitution: Substitution reactions can introduce different functional groups into the this compound molecule.
Common Reagents and Conditions
Common reagents used in these reactions include oxidizing agents like hydrogen peroxide, reducing agents like sodium borohydride, and various catalysts to facilitate substitution reactions. The reaction conditions typically involve controlled temperatures and pH levels to ensure the desired chemical transformations .
Major Products Formed
The major products formed from these reactions include various derivatives of dihydroartemisinin and other artemisinin-related compounds. These products are often used in further research and development of new therapeutic agents .
Scientific Research Applications
Antimalarial Activity
Artesunate is widely recognized for its potent antimalarial properties. The incorporation of deuterium in artesunate-d4 has been shown to influence its pharmacokinetics and efficacy. Studies indicate that this compound retains significant antimalarial activity, similar to its parent compound, while providing advantages in tracking and quantification in biological systems.
- In Vivo Studies : Research demonstrated that this compound exhibited effective antimalarial activity in murine models, with complete cures observed at specific dosages. For example, artesunate administered at 15 mg/kg via intraperitoneal route resulted in a complete cure without recrudescence in mice .
Pharmacokinetic Studies
The use of deuterated compounds like this compound facilitates more accurate pharmacokinetic profiling due to their distinct mass spectrometric signatures. This application is critical for understanding the absorption, distribution, metabolism, and excretion (ADME) of the drug.
- Liquid Chromatography-Mass Spectrometry : A study developed a sensitive liquid chromatography-tandem mass spectrometry method for simultaneous determination of artesunate and amodiaquine using this compound as an internal standard. This method demonstrated high specificity and sensitivity, enabling real-time monitoring of drug levels in plasma samples .
Cancer Therapeutics
Recent studies have explored the potential of artesunate and its derivatives, including this compound, in cancer treatment. Artesunate has shown promise in inducing apoptosis in various cancer cell lines.
- Mechanism of Action : Research indicates that artesunate induces apoptosis through mitochondrial pathways by modulating the expression of pro-apoptotic and anti-apoptotic factors such as Bax and Bcl-2. In gastric cancer cells, treatment with artesunate resulted in significant inhibition of cell proliferation and increased apoptosis rates .
Combination Therapies
This compound has been investigated as part of combination therapies to enhance therapeutic efficacy against malaria and cancer.
- Synergistic Effects : Studies have shown that combining artesunate with other chemotherapeutic agents can lead to enhanced cytotoxic effects. For instance, combined treatment with cisplatin and artesunate resulted in greater tumor growth inhibition compared to either agent alone .
Viral Infections
Emerging research has also suggested potential antiviral applications for artesunate derivatives like this compound.
- SARS-CoV-2 Inhibition : A study evaluated the antiviral activity of artesunate against SARS-CoV-2 in vitro, indicating that combinations with other antimalarials could inhibit viral replication significantly .
Data Table: Summary of Applications
Case Studies
- Antimalarial Efficacy : A study involved administering this compound to mice infected with malaria parasites, demonstrating its effectiveness compared to standard treatments.
- Cancer Treatment : Clinical trials assessing the impact of artesunate on various cancers have shown promising results, particularly in combination therapies where it enhanced the effects of existing chemotherapeutics.
- Viral Activity : In vitro studies highlighted the potential for artesunate derivatives to inhibit viral replication, suggesting avenues for further research into their use against emerging viral threats.
Mechanism of Action
Artesunate-d4, like artesunate, exerts its effects primarily through the generation of reactive oxygen species (ROS) that damage the cellular components of the malaria parasite. The compound targets heme-bound proteins in the parasite’s mitochondria, leading to the inhibition of hemozoin formation and accumulation of toxic heme. This disrupts the parasite’s metabolic processes and ultimately leads to its death .
Comparison with Similar Compounds
Similar Compounds
Artemether: Another artemisinin derivative used in combination with lumefantrine for malaria treatment.
Dihydroartemisinin: The active metabolite of artesunate and other artemisinin derivatives.
Artemisinin: The parent compound from which artesunate and other derivatives are synthesized.
Uniqueness of Artesunate-d4
This compound is unique due to its deuterium labeling, which enhances its stability and allows for precise tracing in analytical studies. This makes it particularly valuable in research settings where accurate quantification and tracking of the compound are essential .
Biological Activity
Artesunate-d4 is a deuterium-labeled derivative of artesunate, a well-known antimalarial compound derived from artemisinin. This modification enhances the pharmacokinetic properties and allows for more precise tracking in biological studies. The biological activity of this compound mirrors that of its parent compound, with additional insights into its mechanisms of action, efficacy against various diseases, and potential therapeutic applications.
Artesunate exhibits a multifaceted mechanism of action, particularly in its role as an antimalarial agent. It primarily functions through the following pathways:
- Inhibition of Heme Polymerization : Artesunate interacts with heme, leading to the generation of reactive oxygen species (ROS), which are toxic to the malaria parasite.
- Alkylation of Proteins : The drug modifies proteins within the parasite, disrupting vital cellular functions.
- Regulation of Apoptosis : Artesunate induces apoptosis in cancer cells by modulating pro-apoptotic and anti-apoptotic factors such as Bax and Bcl-2, leading to mitochondrial dysfunction and caspase activation .
Biological Activities
This compound has demonstrated a range of biological activities beyond its antimalarial effects:
- Anticancer Activity : Studies indicate that artesunate can inhibit the proliferation of various cancer cell lines, including gastric cancer cells. It induces apoptosis in a dose-dependent manner and reduces COX-2 expression, a protein associated with tumor growth .
- Antiviral Properties : Recent research highlights artesunate's potential against viral infections, including its interactions with cytomegalovirus (HCMV) through mitochondrial pathways .
- Anti-inflammatory Effects : Artesunate possesses anti-inflammatory properties that may be beneficial in treating conditions characterized by excessive inflammation .
Efficacy Against Malaria
A pivotal case study illustrated the effectiveness of injectable artesunate in treating severe malaria compared to traditional quinine therapy. The findings indicated a significant reduction in mortality rates among patients treated with artesunate, emphasizing its role as a first-line treatment for severe cases .
Antitumor Activity
In vitro studies have shown that artesunate significantly inhibits the growth of gastric cancer cells. For example, treatment with 20 mg/L artesunate led to a marked reduction in cell viability over 72 hours. Flow cytometry analysis revealed an increase in apoptotic cells from 12.3% to 35.4% upon treatment, indicating strong pro-apoptotic effects .
Data Table: Summary of Biological Activities
Q & A
Basic Research Questions
Q. What are the critical methodological considerations for synthesizing and characterizing Artesunate-d4 in preclinical studies?
- Answer : Synthesis of this compound requires isotopic labeling with deuterium at four specific positions, typically achieved via hydrogen-deuterium exchange under controlled acidic conditions. Characterization should combine nuclear magnetic resonance (NMR) spectroscopy (to confirm deuterium incorporation) and high-resolution mass spectrometry (HRMS) to verify isotopic purity (>98%). Researchers must document reaction conditions (e.g., temperature, solvent, catalyst) and validate reproducibility across batches .
Q. How can researchers optimize liquid chromatography-mass spectrometry (LC-MS) parameters for quantifying this compound in biological matrices?
- Answer : Key parameters include:
- Column selection : Use reverse-phase C18 columns for separation.
- Ionization mode : Electrospray ionization (ESI) in negative mode enhances detection sensitivity.
- Mobile phase : Acetonitrile/ammonium formate buffers (pH 3.5) improve peak resolution.
- Calibration curves : Validate linearity (R² >0.99) across expected concentration ranges (e.g., 1–500 ng/mL in plasma). Include internal standards (e.g., deuterated analogs of related artemisinins) to correct for matrix effects .
Q. What statistical approaches are recommended for analyzing dose-response relationships in this compound efficacy studies?
- Answer : Use nonlinear regression models (e.g., log-logistic or sigmoidal Emax models) to estimate EC50 values. For in vitro assays, normalize data to vehicle controls and apply ANOVA with post-hoc tests (e.g., Tukey’s) to compare treatment groups. Report confidence intervals (95%) and effect sizes to contextualize clinical relevance .
Advanced Research Questions
Q. How should researchers address discrepancies between in vitro and in vivo pharmacokinetic data for this compound?
- Answer : Discrepancies often arise from differences in metabolic stability or protein binding. Mitigate this by:
- Parallel assays : Compare intrinsic clearance rates in hepatocyte incubations (in vitro) with plasma half-life data from rodent models (in vivo).
- Mechanistic modeling : Use physiologically based pharmacokinetic (PBPK) models to simulate absorption-distribution-metabolism-excretion (ADME) processes.
- Tissue sampling : Validate tissue-specific bioavailability via microdialysis or LC-MS imaging .
Q. What experimental designs are optimal for evaluating this compound’s antimalarial resistance mechanisms?
- Answer : Employ a combination of:
- Genomic sequencing : Identify mutations in Plasmodium genes (e.g., pfATP6) associated with reduced drug sensitivity.
- Phenotypic assays : Measure IC50 shifts in synchronized parasite cultures exposed to this compound over multiple generations.
- Proteomics : Quantify expression changes in redox-active proteins (e.g., glutathione S-transferase) via tandem mass tagging (TMT) workflows. Cross-validate findings with isogenic parasite lines .
Q. How can researchers resolve contradictory findings in this compound’s cytotoxic effects on non-target mammalian cells?
- Answer : Contradictions may stem from variability in cell lines or assay conditions. Standardize protocols by:
- Cell line authentication : Use STR profiling to confirm identity.
- Dose calibration : Align treatment durations with physiological exposure times (e.g., 24–72 hours).
- Pathway analysis : Apply RNA-seq or phosphoproteomics to differentiate on-target (e.g., ROS induction) vs. off-target effects. Compare results across multiple labs using shared reference standards .
Q. What strategies improve cross-study comparability when investigating this compound’s metabolite profiles?
- Answer : Harmonize methodologies through:
- Metabolite identification : Use consensus libraries (e.g., HMDB or METLIN) for annotating dihydroartemisinin derivatives.
- Sample preparation : Adopt uniform extraction protocols (e.g., protein precipitation with acetonitrile).
- Data reporting : Follow MIAME/METABOLOMICS standards for metadata, including instrument parameters and QC metrics .
Q. Methodological Guidance for Data Interpretation
Q. How should researchers design controls to isolate this compound’s specific effects in complex biological systems?
- Answer : Include:
- Negative controls : Untreated or vehicle-treated samples.
- Positive controls : Artesunate (non-deuterated) to benchmark deuterium-induced pharmacokinetic changes.
- Isotopic controls : Use analogs labeled at non-critical positions to rule out isotopic interference.
- Knockout models : CRISPR-edited cell lines lacking putative drug targets (e.g., Nrf2 pathway genes) .
Q. What criteria determine the validity of computational models predicting this compound’s binding affinities to hemoglobin degradation products?
- Answer : Validate models using:
- Experimental docking data : Compare in silico predictions with surface plasmon resonance (SPR) or isothermal titration calorimetry (ITC) results.
- Free energy calculations : Apply molecular dynamics (MD) simulations with explicit solvent models.
- Statistical metrics : Calculate root-mean-square deviation (RMSD) <2.0 Å and correlation coefficients (R >0.7) between predicted and observed binding constants .
Properties
IUPAC Name |
2,2,3,3-tetradeuterio-4-oxo-4-[[(1R,4S,5R,8S,9R,10S,12R,13R)-1,5,9-trimethyl-11,14,15,16-tetraoxatetracyclo[10.3.1.04,13.08,13]hexadecan-10-yl]oxy]butanoic acid | |
---|---|---|
Source | PubChem | |
URL | https://pubchem.ncbi.nlm.nih.gov | |
Description | Data deposited in or computed by PubChem | |
InChI |
InChI=1S/C19H28O8/c1-10-4-5-13-11(2)16(23-15(22)7-6-14(20)21)24-17-19(13)12(10)8-9-18(3,25-17)26-27-19/h10-13,16-17H,4-9H2,1-3H3,(H,20,21)/t10-,11-,12+,13+,16-,17-,18-,19-/m1/s1/i6D2,7D2 | |
Source | PubChem | |
URL | https://pubchem.ncbi.nlm.nih.gov | |
Description | Data deposited in or computed by PubChem | |
InChI Key |
FIHJKUPKCHIPAT-SIUCRFQGSA-N | |
Source | PubChem | |
URL | https://pubchem.ncbi.nlm.nih.gov | |
Description | Data deposited in or computed by PubChem | |
Canonical SMILES |
CC1CCC2C(C(OC3C24C1CCC(O3)(OO4)C)OC(=O)CCC(=O)O)C | |
Source | PubChem | |
URL | https://pubchem.ncbi.nlm.nih.gov | |
Description | Data deposited in or computed by PubChem | |
Isomeric SMILES |
[2H]C([2H])(C(=O)O)C([2H])([2H])C(=O)O[C@H]1[C@@H]([C@@H]2CC[C@H]([C@H]3[C@]24[C@H](O1)O[C@@](CC3)(OO4)C)C)C | |
Source | PubChem | |
URL | https://pubchem.ncbi.nlm.nih.gov | |
Description | Data deposited in or computed by PubChem | |
Molecular Formula |
C19H28O8 | |
Source | PubChem | |
URL | https://pubchem.ncbi.nlm.nih.gov | |
Description | Data deposited in or computed by PubChem | |
Molecular Weight |
388.4 g/mol | |
Source | PubChem | |
URL | https://pubchem.ncbi.nlm.nih.gov | |
Description | Data deposited in or computed by PubChem | |
CAS No. |
1316753-15-7 | |
Record name | ||
Source | CAS Common Chemistry | |
URL | https://commonchemistry.cas.org/detail?cas_rn=1316753-15-7 | |
Description | CAS Common Chemistry is an open community resource for accessing chemical information. Nearly 500,000 chemical substances from CAS REGISTRY cover areas of community interest, including common and frequently regulated chemicals, and those relevant to high school and undergraduate chemistry classes. This chemical information, curated by our expert scientists, is provided in alignment with our mission as a division of the American Chemical Society. | |
Explanation | The data from CAS Common Chemistry is provided under a CC-BY-NC 4.0 license, unless otherwise stated. | |
Disclaimer and Information on In-Vitro Research Products
Please be aware that all articles and product information presented on BenchChem are intended solely for informational purposes. The products available for purchase on BenchChem are specifically designed for in-vitro studies, which are conducted outside of living organisms. In-vitro studies, derived from the Latin term "in glass," involve experiments performed in controlled laboratory settings using cells or tissues. It is important to note that these products are not categorized as medicines or drugs, and they have not received approval from the FDA for the prevention, treatment, or cure of any medical condition, ailment, or disease. We must emphasize that any form of bodily introduction of these products into humans or animals is strictly prohibited by law. It is essential to adhere to these guidelines to ensure compliance with legal and ethical standards in research and experimentation.