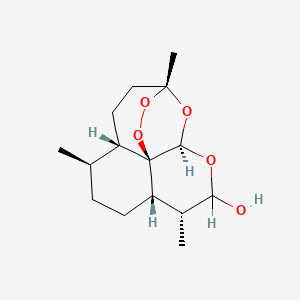
Dihydroartemisinin
Overview
Description
Mechanism of Action
Target of Action
Artenimol, also known as Dihydroartemisinin, is primarily targeted against the Plasmodium falciparum , the parasite responsible for causing malaria. The compound’s action is thought to be mediated through the formation of a reactive carbon radical intermediate, which kills P. falciparum through alkylation of a wide array of proteins .
Mode of Action
Artemisinins, including Artenimol, are thought to act via a common mechanism . The proposed mechanism of action involves cleavage of endoperoxide bridges by iron, producing free radicals (hypervalent iron-oxo species, epoxides, aldehydes, and dicarbonyl compounds) which damage biological macromolecules causing oxidative stress in the cells of the parasite .
Biochemical Pathways
Artenimol is believed to bind to haem produced by the parasite’s haem biosynthesis pathway during the early ring stage of the parasite’s life cycle . In later stages, artemisinins likely bind to haem released by haemoglobin digestion . The activation of Artenimol leads to multiple signaling pathways such as ROS generation, promiscuous protein alkylation, and DNA damage that affect multiple pathways and cellular functions leading to cancer cell death .
Pharmacokinetics
Artenimol exhibits a short half-life and is characterized by rapid action . It is converted primarily to the bioactive metabolite artenimol after either parenteral or gastrointestinal administration . The rate of conversion varies with the route of administration, but the processes need to be characterized further, including the relative contribution of pH and enzymes in tissues, blood, and liver . The oral bioavailability of Artenimol in animals ranges approximately between 19 and 35% .
Result of Action
The main action of Artenimol seems to be directed toward stalling tumor cell proliferation through cell cycle arrest mediated by reactive oxygen species (ROS) . It has been shown to have inhibitory effects against various cancers including pancreatic, osteosarcoma, lung, colon, melanoma, breast, ovarian, prostate, central nervous system, lymphoma, leukemia, and renal .
Action Environment
The yield of Artenimol can be influenced by environmental conditions and field management practices . The use of fertilizer compounds can also affect the Artenimol content . Moreover, the emergence of Artenimol’s nano-based formulations in combination with chemodrugs to enhance drug bioavailability and targeting as well as immunotherapy is also being reviewed .
Biochemical Analysis
Biochemical Properties
Artenimol is thought to form a reactive carbon radical intermediate which kills P. falciparum through alkylation of a wide array of proteins . It interacts with various enzymes, proteins, and other biomolecules, causing oxidative stress in the cells of the parasite .
Cellular Effects
Artenimol has been shown to have anti-cancer properties, stalling tumor cell proliferation through cell cycle arrest mediated by reactive oxygen species . It also exhibits immunosuppressive and anti-inflammatory effects .
Molecular Mechanism
The proposed mechanism of action of Artenimol involves cleavage of endoperoxide bridges by iron, producing free radicals which damage biological macromolecules causing oxidative stress in the cells of the parasite . This reaction is facilitated by the high concentration of free iron in cancer cells .
Preparation Methods
Artenimol is synthesized through the reduction of artemisinin. The process involves the use of sodium borohydride (NaBH4) as a reducing agent in an ethanol solution. The reaction is typically carried out at room temperature, resulting in the formation of dihydroartemisinin . Industrial production methods often involve semi-synthetic routes, where artemisinin is first extracted from Artemisia annua and then chemically modified to produce artenimol .
Chemical Reactions Analysis
Artenimol undergoes several types of chemical reactions, including:
Oxidation: Artenimol can be oxidized to form artemisinin derivatives.
Reduction: The reduction of artemisinin to artenimol is a common reaction.
Substitution: Artenimol can undergo substitution reactions to form various derivatives.
Common reagents used in these reactions include sodium borohydride for reduction and hydrogen peroxide for oxidation . The major products formed from these reactions are typically other artemisinin derivatives, which retain the antimalarial properties of the parent compound .
Scientific Research Applications
Artenimol has a wide range of scientific research applications:
Chemistry: It is used as an intermediate in the synthesis of other artemisinin derivatives.
Biology: Artenimol is studied for its effects on various biological pathways, particularly those related to malaria.
Medicine: Beyond its antimalarial properties, artenimol is being investigated for its potential use in cancer therapy due to its ability to induce oxidative stress in cancer cells
Industry: Artenimol is used in the pharmaceutical industry for the production of antimalarial drugs.
Comparison with Similar Compounds
Artenimol is compared with other artemisinin derivatives such as:
- Artemether
- Artesunate
- Artemisinin
Artenimol is unique due to its high bioavailability and rapid action against malaria parasites . While artemether and artesunate are also effective antimalarial agents, artenimol is often preferred for its ability to quickly reduce parasite load .
Properties
IUPAC Name |
(1R,4S,5R,8S,9R,12R,13R)-1,5,9-trimethyl-11,14,15,16-tetraoxatetracyclo[10.3.1.04,13.08,13]hexadecan-10-ol | |
---|---|---|
Source | PubChem | |
URL | https://pubchem.ncbi.nlm.nih.gov | |
Description | Data deposited in or computed by PubChem | |
InChI |
InChI=1S/C15H24O5/c1-8-4-5-11-9(2)12(16)17-13-15(11)10(8)6-7-14(3,18-13)19-20-15/h8-13,16H,4-7H2,1-3H3/t8-,9-,10+,11+,12?,13-,14-,15-/m1/s1 | |
Source | PubChem | |
URL | https://pubchem.ncbi.nlm.nih.gov | |
Description | Data deposited in or computed by PubChem | |
InChI Key |
BJDCWCLMFKKGEE-HVDUHBCDSA-N | |
Source | PubChem | |
URL | https://pubchem.ncbi.nlm.nih.gov | |
Description | Data deposited in or computed by PubChem | |
Canonical SMILES |
CC1CCC2C(C(OC3C24C1CCC(O3)(OO4)C)O)C | |
Source | PubChem | |
URL | https://pubchem.ncbi.nlm.nih.gov | |
Description | Data deposited in or computed by PubChem | |
Isomeric SMILES |
C[C@@H]1CC[C@H]2[C@H](C(O[C@H]3[C@@]24[C@H]1CC[C@](O3)(OO4)C)O)C | |
Source | PubChem | |
URL | https://pubchem.ncbi.nlm.nih.gov | |
Description | Data deposited in or computed by PubChem | |
Molecular Formula |
C15H24O5 | |
Source | PubChem | |
URL | https://pubchem.ncbi.nlm.nih.gov | |
Description | Data deposited in or computed by PubChem | |
Molecular Weight |
284.35 g/mol | |
Source | PubChem | |
URL | https://pubchem.ncbi.nlm.nih.gov | |
Description | Data deposited in or computed by PubChem | |
Mechanism of Action |
Artemisinins, including Artenimol which is a major active metabolite of many artemisinins, are thought to act via a common mechanism. While the exact mechanism of action is not certain, theories exist as to how artemisinins produce their antimalarial effect. Artemisinins are believed to bind to haem within the *P. falciparum* parasite. The source of this haem varies with the life stage of the parasite. When the parasite is in the early ring stage artemisinins are believed to bind haem produced by the parasite's haem biosynthesis pathway. In later stages artemisinins likely bind to haem released by haemoglobin digestion. Once bound to haem, artemisinins are thought to undergo activation involving ferrous iron via reductive scission which splits the endoperoxide bridge to produce a reactive oxygen. This reactive oxygen is thought to undergo a subsequent intramolecular hydrogen abstraction to produce a reactive carbon radical. The carbon radical is believed to be the source of the drugs potent activity against *P. falciparum* by alkylating a wide array of protein targets. The nature and magnitude of the effect on specific protein function as a result of this alkylation is unknown. One target which has been the focus of research is the sarco/endoplasmic reticulum Ca2+ ATPase pump of *P. falciparum*. Artemisinins have been found to irreversably bind to and inhibit this protein at a binding site similar to that of Thapsigargin. The mechanism is likely the same as for other proteins, namely alkylation via the carbon radical intermediate. Artemisinins appear to preferentially collect in infected erythrocytes, concentrating the drug by several hundred-fold compared to uninfected cells. This may play a role in why little alkylation is seen in uninfected erythrocytes. | |
Record name | Artenimol | |
Source | DrugBank | |
URL | https://www.drugbank.ca/drugs/DB11638 | |
Description | The DrugBank database is a unique bioinformatics and cheminformatics resource that combines detailed drug (i.e. chemical, pharmacological and pharmaceutical) data with comprehensive drug target (i.e. sequence, structure, and pathway) information. | |
Explanation | Creative Common's Attribution-NonCommercial 4.0 International License (http://creativecommons.org/licenses/by-nc/4.0/legalcode) | |
CAS No. |
71939-50-9 | |
Record name | Artenimol | |
Source | DrugBank | |
URL | https://www.drugbank.ca/drugs/DB11638 | |
Description | The DrugBank database is a unique bioinformatics and cheminformatics resource that combines detailed drug (i.e. chemical, pharmacological and pharmaceutical) data with comprehensive drug target (i.e. sequence, structure, and pathway) information. | |
Explanation | Creative Common's Attribution-NonCommercial 4.0 International License (http://creativecommons.org/licenses/by-nc/4.0/legalcode) | |
Melting Point |
164-165 | |
Record name | Artenimol | |
Source | DrugBank | |
URL | https://www.drugbank.ca/drugs/DB11638 | |
Description | The DrugBank database is a unique bioinformatics and cheminformatics resource that combines detailed drug (i.e. chemical, pharmacological and pharmaceutical) data with comprehensive drug target (i.e. sequence, structure, and pathway) information. | |
Explanation | Creative Common's Attribution-NonCommercial 4.0 International License (http://creativecommons.org/licenses/by-nc/4.0/legalcode) | |
Disclaimer and Information on In-Vitro Research Products
Please be aware that all articles and product information presented on BenchChem are intended solely for informational purposes. The products available for purchase on BenchChem are specifically designed for in-vitro studies, which are conducted outside of living organisms. In-vitro studies, derived from the Latin term "in glass," involve experiments performed in controlled laboratory settings using cells or tissues. It is important to note that these products are not categorized as medicines or drugs, and they have not received approval from the FDA for the prevention, treatment, or cure of any medical condition, ailment, or disease. We must emphasize that any form of bodily introduction of these products into humans or animals is strictly prohibited by law. It is essential to adhere to these guidelines to ensure compliance with legal and ethical standards in research and experimentation.