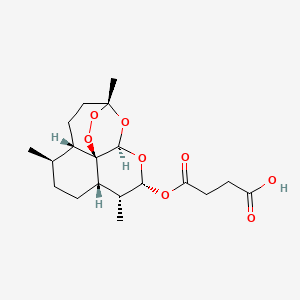
Artesunate
Overview
Description
Artesunate is a semi-synthetic derivative of artemisinin, a sesquiterpene lactone isolated from the plant Artemisia annua. It is a cornerstone of artemisinin-based combination therapies (ACTs) for uncomplicated Plasmodium falciparum malaria due to its rapid parasiticidal activity and favorable safety profile . This compound’s mechanism involves the cleavage of its endoperoxide bridge by intraparasitic iron, generating reactive oxygen species (ROS) that damage parasitic proteins and membranes . Beyond antimalarial use, it exhibits anticancer, immunomodulatory, and herbicidal properties .
Preparation Methods
Synthetic Routes and Reaction Conditions: Artesunate is synthesized from artemisinin through a two-step process. The first step involves the reduction of artemisinin to dihydroartemisinin using sodium borohydride (NaBH4). The second step is the esterification of dihydroartemisinin with succinic anhydride under basic conditions . This method is efficient and follows green chemistry principles, utilizing reusable solvents .
Industrial Production Methods: On an industrial scale, the production of this compound involves the isolation of artemisinin from the dried leaves of Artemisia annua. The isolated artemisinin is then converted to dihydroartemisinin and subsequently to this compound using the aforementioned synthetic route .
Chemical Reactions Analysis
Hydrolysis to Dihydroartemisinin (DHA)
Artesunate undergoes rapid hydrolysis via plasma esterases to form its active metabolite, dihydroartemisinin (DHA) . This reaction is pH- and temperature-dependent, following pseudo-first-order kinetics :
Hydrolysis Reaction:
Temperature (°C) | Rate Constant (k, h⁻¹) | Half-Life (t₁/₂) | Shelf-Life (t₀.₉) |
---|---|---|---|
40 | 0.056 | 12.4 h | 1.8 h |
25 | 0.006 | 114.6 h | 17.3 h |
5 | 0.00066 | 1053.4 h | 158.9 h |
The activation energy (Eₐ) for hydrolysis is 90.84 kJ/mol , with a frequency factor (A) of 6.67 × 10¹³ h⁻¹ . Buffer strength and mannitol concentration modulate degradation rates.
Heme Alkylation in Malaria Parasites
The antimalarial mechanism involves alkylation of heme via free radicals generated from the endoperoxide bridge :
Reaction Pathway:
-
Iron-Mediated Activation:
Lysosomal iron in Plasmodium reduces the endoperoxide bridge, producing carbon-centered radicals . -
Covalent Modification:
Radicals alkylate heme at meso-positions, forming covalent adducts that disrupt hemozoin formation .
DFT Analysis of Heme-DHA Adducts :
Adduct Type | Bond Length (Å) | Out-of-Plane Displacement (Å) | Energy (kcal/mol) |
---|---|---|---|
Mono-meso-C10 | 1.54 | 0.12 | -152.3 |
Di-meso-C10/C15 | 1.52 | 0.18 | -148.7 |
Alkylation distorts heme planarity, inhibiting detoxification and inducing oxidative stress .
Reactive Oxygen Species (ROS) Generation
This compound’s endoperoxide moiety generates ROS through iron-catalyzed Fenton reactions :
-
Parasitic Proteins: Alkylation of falcipain-2 (cysteine protease) and EXP1 (glutathione S-transferase).
-
Mitochondrial Dysfunction: ROS oxidize mitochondrial membranes, releasing pro-apoptotic factors .
Degradation in Aqueous Solutions
This compound degrades via ester hydrolysis under alkaline conditions, forming DHA and succinate :
Degradation Profile:
Parameter | Value |
---|---|
Optimal pH | 9.0 (phosphate buffer) |
Activation Energy | 90.84 kJ/mol |
Frequency Factor | 6.67 × 10¹³ h⁻¹ |
Shelf-Life (30°C) | 7.2 h |
Mannitol stabilizes this compound by scavenging free radicals, extending shelf-life .
Synthetic Derivatization
This compound is synthesized from dihydroartemisinin (DHA) via esterification with succinic anhydride :
Reaction:
Key Features:
-
Water-soluble due to the succinate ester group.
Lysosomal Iron-Mediated Cytotoxicity in Cancer
In cancer cells, lysosomal iron catalyzes this compound’s activation, producing ROS and inducing ferroptosis :
Mechanistic Steps:
-
Lysosomal Accumulation: this compound localizes in lysosomes rich in redox-active iron.
-
ROS Burst: Iron reduces the endoperoxide bridge, generating hydroxyl radicals.
-
Membrane Permeabilization: Lysosomal rupture releases cathepsins, triggering mitochondrial apoptosis .
Selectivity: Cancer cells’ elevated iron uptake enhances this compound’s cytotoxicity .
Interaction with Serum Proteins
This compound binds to serum albumin (log Kₐ = 4.2), altering its pharmacokinetics . Competitive binding with fatty acids reduces free drug availability .
Scientific Research Applications
Antimalarial Properties
Primary Use in Malaria Treatment
Artesunate is widely recognized as a first-line treatment for malaria, particularly severe cases caused by Plasmodium falciparum. Its efficacy is attributed to its rapid action against the parasite and its ability to reduce gametocyte carriage, thereby decreasing transmission rates. This compound is often combined with longer-acting partner drugs in artemisinin-based combination therapies (ACTs), which are essential in malaria control strategies across endemic regions .
Anticancer Activity
Mechanisms of Action
Recent studies have highlighted this compound's potential as an anticancer agent. It has been shown to induce apoptosis in various cancer cell lines through several mechanisms:
- Reactive Oxygen Species (ROS) Generation : this compound promotes oxidative stress in tumor cells, leading to DNA damage and apoptosis .
- Inhibition of Tumor Growth : It has demonstrated anti-angiogenic properties, which hinder tumor blood supply and growth .
- Ferroptosis Induction : this compound can trigger ferroptosis, a form of programmed cell death linked to iron metabolism, providing a novel approach to cancer therapy .
Case Studies
Clinical trials have reported promising results in treating cancers such as breast cancer and leukemia. For instance, this compound has been involved in studies where it showed significant tumor reduction in patients resistant to conventional therapies .
Neurological Disorders
Therapeutic Potential
this compound's ability to penetrate the blood-brain barrier presents opportunities for treating neurological disorders. Research indicates that it can protect against secondary brain injuries following strokes and traumatic brain injuries by:
Animal Studies
Preclinical models have demonstrated that this compound administration post-injury can improve neurological outcomes significantly, suggesting its potential role in managing conditions like subarachnoid hemorrhage and ischemic strokes .
Antiviral Activity
Broad-Spectrum Antiviral Effects
Emerging evidence suggests that this compound exhibits antiviral properties against various viruses, including coronaviruses. Its mechanism involves disrupting viral replication and modulating immune responses . Notably, during the COVID-19 pandemic, studies explored its efficacy as an adjunctive therapy due to its immunomodulatory effects.
Other Therapeutic Applications
Anti-inflammatory and Immunosuppressive Effects
this compound has shown promise in treating inflammatory conditions by modulating cytokine production and reducing inflammation markers. This property could be beneficial for autoimmune diseases and chronic inflammatory disorders .
Summary Table of Applications
Application Area | Mechanism of Action | Evidence Level |
---|---|---|
Antimalarial | Rapid parasite clearance, reduction of gametocyte carriage | High |
Anticancer | Induces apoptosis via ROS generation | Moderate to High |
Neurological Disorders | Protects BBB integrity, reduces neuroinflammation | Moderate |
Antiviral | Disrupts viral replication | Emerging |
Anti-inflammatory | Modulates cytokine production | Moderate |
Mechanism of Action
Artesunate is metabolized to dihydroartemisinin, which generates free radicals through the cleavage of its endoperoxide bridge. These free radicals inhibit the synthesis of proteins and nucleic acids in Plasmodium parasites, leading to their death . The primary molecular targets are the parasitized erythrocyte membranes, where the free radicals accumulate and exert their toxic effects .
Comparison with Similar Compounds
Artemisinin Derivatives
Artesunate is compared with other artemisinin derivatives, including artemether , dihydroartemisinin (DHA) , and structural analogs.
IC50 values against *P. falciparum in vitro .
- Efficacy : this compound and DHA exhibit nearly identical antimalarial potency, with geometric mean IC50 values of 2.1 nM and 1.9 nM, respectively, in Thai P. falciparum isolates .
- Safety : Intramuscular artemether shows higher neurotoxicity in experimental mammals than this compound, likely due to prolonged tissue retention .
Structural Analogs and Hybrids
Modified this compound derivatives aim to enhance bioavailability or overcome resistance:
- Mechanistic Insights : this compound dimers and polyamine conjugates retain the critical endoperoxide bridge, ensuring ROS generation . Hybrids like this compound-azadirachtin exploit structural similarities to target parasitic proteins like gephyrin .
Non-Artemisinin Antimalarials
Compared to slower-acting antimalarials:
Compound | Parasite Reduction Ratio (PRR)* | Speed of Action | Resistance Risk |
---|---|---|---|
This compound | 87–93% (24 h) | Fast | Low |
Atovaquone | 40–60% (24 h) | Slow | High |
Pyrimethamine | 50–70% (24 h) | Moderate | High |
PRR in *P. falciparum cultures .
- Combination Therapy : this compound synergizes with lumefantrine (ACT partner) but shows antagonism with certain Malaria Box compounds (e.g., compounds 1, 3–8, 11) .
Herbicidal and Anticancer Compounds
This compound’s off-target effects highlight overlaps with non-antimalarial agents:
Herbicidal activity in *A. thaliana .
- Anticancer Activity : this compound inhibits Burkitt’s lymphoma proliferation via caspase-9/3 activation (IC50: 10–20 μM) and induces G2/M arrest in leukemia cells .
Biological Activity
Artesunate, a semisynthetic derivative of artemisinin, is primarily recognized for its efficacy in treating malaria. However, its biological activity extends beyond this application, demonstrating significant effects against various cancers, viral infections, and other health conditions. This article delves into the mechanisms of action, therapeutic applications, and recent research findings related to this compound.
This compound exerts its biological effects through several mechanisms:
- Antimalarial Activity : this compound's primary mechanism involves the generation of reactive oxygen species (ROS) upon interaction with heme. This process leads to oxidative damage to the malaria parasite, ultimately resulting in its death. The endoperoxide moiety of this compound is crucial for this activity, as it activates upon contact with iron within the parasite .
-
Anticancer Properties : Recent studies have highlighted this compound's potential in oncology. It induces cell cycle arrest and apoptosis in cancer cells through various pathways:
- Autophagy : this compound can trigger autophagic processes that lead to non-apoptotic cell death.
- Ferroptosis : This form of regulated cell death is induced by oxidative stress and is influenced by this compound's ability to generate ROS.
- Inhibition of Angiogenesis : this compound has been shown to inhibit blood vessel formation in tumors, thereby limiting their growth and metastasis .
- Antiviral Effects : Research indicates that this compound may inhibit the replication of certain viruses, including human cytomegalovirus (HCMV), by disrupting mitochondrial functions and NF-κB signaling pathways .
Therapeutic Applications
This compound's broad spectrum of biological activity has led to its investigation for various therapeutic uses:
- Malaria Treatment : this compound is recommended by the World Health Organization (WHO) as a first-line treatment for severe malaria due to its rapid action and reduced mortality rates compared to traditional treatments like quinine. A meta-analysis showed a significant reduction in mortality rates among patients treated with this compound .
- Cancer Therapy : Clinical studies are exploring the use of this compound in combination with other chemotherapeutic agents for enhanced anticancer efficacy. It has been shown to inhibit tumor growth in various cancer models .
- Inflammatory Diseases : this compound exhibits anti-inflammatory properties, which may be beneficial in treating conditions like rheumatoid arthritis and inflammatory bowel diseases .
Recent Research Findings
Recent studies have provided insights into the pharmacological profile and efficacy of this compound:
- Clinical Trials : A large-scale trial demonstrated that this compound significantly reduces mortality rates in children with severe malaria by 22.5% compared to quinine. In adults, a similar study indicated a 35% reduction in death rates when this compound was used .
- Pharmacokinetics : The bioavailability of this compound has been assessed, revealing a mean oral bioavailability of approximately 61% during acute malaria episodes. The rapid absorption and elimination profile supports its use as an effective treatment option .
Efficacy Comparison of this compound vs. Quinine
Treatment | Mortality Rate (%) | Relative Reduction (%) | Study Population |
---|---|---|---|
This compound | 8.5 | 22.5 | African children |
Quinine | 10.9 | - | African children |
This compound | 14.2 | 38.6 | Asian adults |
Quinine | 23.1 | - | Asian adults |
Summary of Biological Activities
Biological Activity | Mechanism/Effect |
---|---|
Antimalarial | ROS generation, heme interaction |
Anticancer | Induction of apoptosis, inhibition of angiogenesis |
Antiviral | Disruption of viral replication pathways |
Anti-inflammatory | Reduction of inflammatory cytokines |
Case Studies
- Severe Malaria Treatment : In a double-blind trial involving 370 patients with severe falciparum malaria, those treated with this compound had a significantly lower mortality rate compared to those receiving quinine (8.5% vs. 10.9%) .
- Cancer Treatment : A study on non-small cell lung cancer cells demonstrated that this compound inhibited cell proliferation and induced apoptosis through ROS-mediated pathways .
Q & A
Basic Research Questions
Q. What experimental protocols are recommended for assessing Artesunate’s antimalarial efficacy in vitro?
Methodological Answer: Standardized protocols involve dose-response assays using synchronized Plasmodium falciparum cultures. Fluorescence-based assays (e.g., SYBR Green I) quantify parasite viability post-treatment. Include controls for hemolysis and solvent toxicity (e.g., DMSO). Data should be analyzed via nonlinear regression models (e.g., four-parameter logistic curves) to calculate IC50 values .
Q. How do researchers validate this compound’s pharmacokinetic properties in preclinical models?
Methodological Answer: Employ high-performance liquid chromatography (HPLC) or LC-MS/MS to measure this compound and its active metabolite, dihydroartemisinin, in plasma/tissue samples. Use compartmental modeling (e.g., non-linear mixed-effects) to estimate parameters like clearance and volume of distribution. Ensure species-specific metabolic differences are accounted for in extrapolation to humans .
Q. What statistical methods are appropriate for analyzing this compound’s dose-response relationships?
Methodological Answer: Four-parameter nonlinear regression is standard for generating inhibition curves. Log-transformation of concentration data improves linearity visualization. Report variability using standard deviation (SD) or confidence intervals (CI) across independent replicates (≥9 assays recommended for robustness) .
Advanced Research Questions
Q. How can researchers address contradictory findings in this compound’s modulation of host signaling pathways (e.g., cAMP vs. Wnt/β-catenin)?
Methodological Answer: Contradictions may arise from cell-type specificity or concentration-dependent effects. Use transcriptomic profiling (e.g., microarrays) combined with pathway enrichment analysis (e.g., Ingenuity Pathway Analysis) to identify context-dependent regulatory mechanisms. Validate findings via CRISPR/Cas9-mediated gene silencing or pharmacological inhibitors .
Q. What strategies mitigate variability in this compound’s parasite growth inhibition assays?
Methodological Answer: Standardize parasite synchronization (e.g., sorbitol lysis) and culture conditions (e.g., hematocrit, serum batches). Include intra-assay controls and normalize data to baseline fluorescence. For cross-study comparisons, use meta-analytical approaches to aggregate data and adjust for batch effects .
Q. How should researchers design studies to investigate this compound’s off-target effects in non-malarial cells?
Methodological Answer: Conduct cytotoxicity screens across diverse cell lines (e.g., RAW 264.7 macrophages, HepG2 hepatocytes) using MTT or Annexin V assays. Pair with multi-omics approaches (proteomics/metabolomics) to distinguish specific vs. bystander effects. Dose ranges should mirror therapeutic plasma levels to ensure clinical relevance .
Q. What experimental controls are critical for evaluating this compound’s immunomodulatory roles?
Methodological Answer: Include LPS-stimulated positive controls and unstimulated negative controls in cytokine assays (e.g., ELISA). Use isotype controls in flow cytometry to gate immune cell populations. For in vivo studies, employ knockout models (e.g., iNOS<sup>-/-</sup> mice) to dissect nitric oxide-dependent mechanisms .
Q. Data Analysis & Interpretation
Q. How can researchers reconcile discrepancies between in vitro and in vivo efficacy data for this compound?
Methodological Answer: Perform pharmacokinetic-pharmacodynamic (PK/PD) modeling to account for bioavailability and tissue penetration differences. Use ex vivo assays (e.g., ex vivo parasite inhibition in patient-derived RBCs) as a translational bridge. Validate findings with clinical PK/PD correlations from Phase II trials .
Q. What bioinformatic tools are recommended for analyzing this compound-induced gene expression changes?
Methodological Answer: Use DESeq2 or edgeR for differential expression analysis of RNA-seq data. Pathway mapping tools like DAVID or GSEA identify enriched biological processes. For network analysis, employ Cytoscape with plugins (e.g., ClueGO) to visualize gene interactions .
Q. Experimental Design Challenges
Q. How do researchers ensure reproducibility in this compound studies given batch-to-batch compound variability?
Methodological Answer: Source this compound from certified suppliers (e.g., WHO-prequalified). Validate purity via NMR or mass spectrometry. Include reference standards in each experiment and report batch numbers in publications. Collaborative inter-laboratory studies can benchmark variability thresholds .
Q. What ethical considerations apply when designing human trials for this compound combination therapies?
Methodological Answer: Adhere to CONSORT guidelines for randomized controlled trials. Include data safety monitoring boards (DSMBs) to review adverse events. Obtain informed consent with explicit disclosure of this compound’s short half-life and potential recrudescence risks .
Q. Tables for Key Data
Table 1. this compound-Induced Gene Expression in RAW 264.7 Macrophages
Gene Symbol | Fold Change | Pathway Association |
---|---|---|
Pde4b | +3.2 | cAMP Signaling |
Axin2 | -2.1 | Wnt/β-catenin |
Table 2. Variability in IC50 Values Across Plasmodium Strains
Strain | IC50 (nM) | SD |
---|---|---|
3D7 | 6.8 | ±1.2 |
Dd2 | 12.4 | ±2.3 |
Properties
IUPAC Name |
4-oxo-4-[[(1R,4S,5R,8S,9R,10R,12R,13R)-1,5,9-trimethyl-11,14,15,16-tetraoxatetracyclo[10.3.1.04,13.08,13]hexadecan-10-yl]oxy]butanoic acid | |
---|---|---|
Details | Computed by Lexichem TK 2.7.0 (PubChem release 2021.05.07) | |
Source | PubChem | |
URL | https://pubchem.ncbi.nlm.nih.gov | |
Description | Data deposited in or computed by PubChem | |
InChI |
InChI=1S/C19H28O8/c1-10-4-5-13-11(2)16(23-15(22)7-6-14(20)21)24-17-19(13)12(10)8-9-18(3,25-17)26-27-19/h10-13,16-17H,4-9H2,1-3H3,(H,20,21)/t10-,11-,12+,13+,16+,17-,18-,19-/m1/s1 | |
Details | Computed by InChI 1.0.6 (PubChem release 2021.05.07) | |
Source | PubChem | |
URL | https://pubchem.ncbi.nlm.nih.gov | |
Description | Data deposited in or computed by PubChem | |
InChI Key |
FIHJKUPKCHIPAT-NKHDUEHSSA-N | |
Details | Computed by InChI 1.0.6 (PubChem release 2021.05.07) | |
Source | PubChem | |
URL | https://pubchem.ncbi.nlm.nih.gov | |
Description | Data deposited in or computed by PubChem | |
Canonical SMILES |
CC1CCC2C(C(OC3C24C1CCC(O3)(OO4)C)OC(=O)CCC(=O)O)C | |
Details | Computed by OEChem 2.3.0 (PubChem release 2021.05.07) | |
Source | PubChem | |
URL | https://pubchem.ncbi.nlm.nih.gov | |
Description | Data deposited in or computed by PubChem | |
Isomeric SMILES |
C[C@@H]1CC[C@H]2[C@H]([C@H](O[C@H]3[C@@]24[C@H]1CC[C@](O3)(OO4)C)OC(=O)CCC(=O)O)C | |
Details | Computed by OEChem 2.3.0 (PubChem release 2021.05.07) | |
Source | PubChem | |
URL | https://pubchem.ncbi.nlm.nih.gov | |
Description | Data deposited in or computed by PubChem | |
Molecular Formula |
C19H28O8 | |
Details | Computed by PubChem 2.1 (PubChem release 2021.05.07) | |
Source | PubChem | |
URL | https://pubchem.ncbi.nlm.nih.gov | |
Description | Data deposited in or computed by PubChem | |
DSSTOX Substance ID |
DTXSID30861106 | |
Record name | beta-Artesunate | |
Source | EPA DSSTox | |
URL | https://comptox.epa.gov/dashboard/DTXSID30861106 | |
Description | DSSTox provides a high quality public chemistry resource for supporting improved predictive toxicology. | |
Molecular Weight |
384.4 g/mol | |
Details | Computed by PubChem 2.1 (PubChem release 2021.05.07) | |
Source | PubChem | |
URL | https://pubchem.ncbi.nlm.nih.gov | |
Description | Data deposited in or computed by PubChem | |
Color/Form |
Fine white crystalline powder | |
CAS No. |
182824-33-5, 88495-63-0 | |
Record name | 1-[(3R,5aS,6R,8aS,9R,10R,12R,12aR)-Decahydro-3,6,9-trimethyl-3,12-epoxy-12H-pyrano[4,3-j]-1,2-benzodioxepin-10-yl] butanedioate | |
Source | CAS Common Chemistry | |
URL | https://commonchemistry.cas.org/detail?cas_rn=182824-33-5 | |
Description | CAS Common Chemistry is an open community resource for accessing chemical information. Nearly 500,000 chemical substances from CAS REGISTRY cover areas of community interest, including common and frequently regulated chemicals, and those relevant to high school and undergraduate chemistry classes. This chemical information, curated by our expert scientists, is provided in alignment with our mission as a division of the American Chemical Society. | |
Explanation | The data from CAS Common Chemistry is provided under a CC-BY-NC 4.0 license, unless otherwise stated. | |
Record name | beta-Artesunate | |
Source | ChemIDplus | |
URL | https://pubchem.ncbi.nlm.nih.gov/substance/?source=chemidplus&sourceid=0182824335 | |
Description | ChemIDplus is a free, web search system that provides access to the structure and nomenclature authority files used for the identification of chemical substances cited in National Library of Medicine (NLM) databases, including the TOXNET system. | |
Record name | beta-Artesunate | |
Source | EPA DSSTox | |
URL | https://comptox.epa.gov/dashboard/DTXSID30861106 | |
Description | DSSTox provides a high quality public chemistry resource for supporting improved predictive toxicology. | |
Record name | Butanedioic acid, 1-[(3R,5aS,6R,8aS,9R,10R,12R,12aR)-decahydro-3,6,9-trimethyl-3,12-epoxy-12H-pyrano[4,3-j]-1,2-benzodioxepin-10-yl] ester | |
Source | European Chemicals Agency (ECHA) | |
URL | https://echa.europa.eu/substance-information/-/substanceinfo/100.128.794 | |
Description | The European Chemicals Agency (ECHA) is an agency of the European Union which is the driving force among regulatory authorities in implementing the EU's groundbreaking chemicals legislation for the benefit of human health and the environment as well as for innovation and competitiveness. | |
Explanation | Use of the information, documents and data from the ECHA website is subject to the terms and conditions of this Legal Notice, and subject to other binding limitations provided for under applicable law, the information, documents and data made available on the ECHA website may be reproduced, distributed and/or used, totally or in part, for non-commercial purposes provided that ECHA is acknowledged as the source: "Source: European Chemicals Agency, http://echa.europa.eu/". Such acknowledgement must be included in each copy of the material. ECHA permits and encourages organisations and individuals to create links to the ECHA website under the following cumulative conditions: Links can only be made to webpages that provide a link to the Legal Notice page. | |
Record name | Butanedioic acid, 1-[(3R,5aS,6R,8aS,9R,10S,12R,12aR)-decahydro-3,6,9-trimethyl-3,12-epoxy-12H-pyrano[4,3-j]-1,2-benzodioxepin-10-yl] ester | |
Source | European Chemicals Agency (ECHA) | |
URL | https://echa.europa.eu/substance-information/-/substanceinfo/100.106.898 | |
Description | The European Chemicals Agency (ECHA) is an agency of the European Union which is the driving force among regulatory authorities in implementing the EU's groundbreaking chemicals legislation for the benefit of human health and the environment as well as for innovation and competitiveness. | |
Explanation | Use of the information, documents and data from the ECHA website is subject to the terms and conditions of this Legal Notice, and subject to other binding limitations provided for under applicable law, the information, documents and data made available on the ECHA website may be reproduced, distributed and/or used, totally or in part, for non-commercial purposes provided that ECHA is acknowledged as the source: "Source: European Chemicals Agency, http://echa.europa.eu/". Such acknowledgement must be included in each copy of the material. ECHA permits and encourages organisations and individuals to create links to the ECHA website under the following cumulative conditions: Links can only be made to webpages that provide a link to the Legal Notice page. | |
Record name | .BETA.-ARTESUNATE | |
Source | FDA Global Substance Registration System (GSRS) | |
URL | https://gsrs.ncats.nih.gov/ginas/app/beta/substances/USD5X0Z99I | |
Description | The FDA Global Substance Registration System (GSRS) enables the efficient and accurate exchange of information on what substances are in regulated products. Instead of relying on names, which vary across regulatory domains, countries, and regions, the GSRS knowledge base makes it possible for substances to be defined by standardized, scientific descriptions. | |
Explanation | Unless otherwise noted, the contents of the FDA website (www.fda.gov), both text and graphics, are not copyrighted. They are in the public domain and may be republished, reprinted and otherwise used freely by anyone without the need to obtain permission from FDA. Credit to the U.S. Food and Drug Administration as the source is appreciated but not required. | |
Record name | ARTESUNIC ACID | |
Source | Hazardous Substances Data Bank (HSDB) | |
URL | https://pubchem.ncbi.nlm.nih.gov/source/hsdb/7458 | |
Description | The Hazardous Substances Data Bank (HSDB) is a toxicology database that focuses on the toxicology of potentially hazardous chemicals. It provides information on human exposure, industrial hygiene, emergency handling procedures, environmental fate, regulatory requirements, nanomaterials, and related areas. The information in HSDB has been assessed by a Scientific Review Panel. | |
Retrosynthesis Analysis
AI-Powered Synthesis Planning: Our tool employs the Template_relevance Pistachio, Template_relevance Bkms_metabolic, Template_relevance Pistachio_ringbreaker, Template_relevance Reaxys, Template_relevance Reaxys_biocatalysis model, leveraging a vast database of chemical reactions to predict feasible synthetic routes.
One-Step Synthesis Focus: Specifically designed for one-step synthesis, it provides concise and direct routes for your target compounds, streamlining the synthesis process.
Accurate Predictions: Utilizing the extensive PISTACHIO, BKMS_METABOLIC, PISTACHIO_RINGBREAKER, REAXYS, REAXYS_BIOCATALYSIS database, our tool offers high-accuracy predictions, reflecting the latest in chemical research and data.
Strategy Settings
Precursor scoring | Relevance Heuristic |
---|---|
Min. plausibility | 0.01 |
Model | Template_relevance |
Template Set | Pistachio/Bkms_metabolic/Pistachio_ringbreaker/Reaxys/Reaxys_biocatalysis |
Top-N result to add to graph | 6 |
Feasible Synthetic Routes
Disclaimer and Information on In-Vitro Research Products
Please be aware that all articles and product information presented on BenchChem are intended solely for informational purposes. The products available for purchase on BenchChem are specifically designed for in-vitro studies, which are conducted outside of living organisms. In-vitro studies, derived from the Latin term "in glass," involve experiments performed in controlled laboratory settings using cells or tissues. It is important to note that these products are not categorized as medicines or drugs, and they have not received approval from the FDA for the prevention, treatment, or cure of any medical condition, ailment, or disease. We must emphasize that any form of bodily introduction of these products into humans or animals is strictly prohibited by law. It is essential to adhere to these guidelines to ensure compliance with legal and ethical standards in research and experimentation.