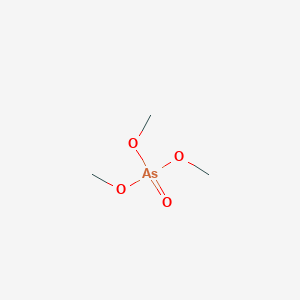
Trimethyl arsenate
Overview
Description
Sophoraflavanone B, also known as 8-prenylnaringenin, is a prenylflavonoid phytoestrogen. It is a naturally occurring compound found in the roots of Sophora flavescens, a plant widely distributed in Asia. This compound is known for its strong estrogenic activity and has been studied for its potential therapeutic applications .
Preparation Methods
Synthetic Routes and Reaction Conditions
Sophoraflavanone B can be synthesized through various chemical reactions. One common method involves the prenylation of naringenin. The reaction typically requires a prenyl donor, such as prenyl bromide, and a base, such as potassium carbonate, in an organic solvent like acetone. The reaction is carried out under reflux conditions to yield sophoraflavanone B .
Industrial Production Methods
Industrial production of sophoraflavanone B often involves the extraction from natural sources, such as the roots of Sophora flavescens. The extraction process includes drying and grinding the plant material, followed by solvent extraction using methanol or ethanol. The extract is then purified using chromatographic techniques to isolate sophoraflavanone B .
Chemical Reactions Analysis
Types of Reactions
Sophoraflavanone B undergoes various chemical reactions, including:
Oxidation: It can be oxidized to form different quinones.
Reduction: Reduction reactions can convert it into dihydro derivatives.
Substitution: It can undergo nucleophilic substitution reactions, particularly at the prenyl group.
Common Reagents and Conditions
Oxidation: Common oxidizing agents include potassium permanganate and hydrogen peroxide.
Reduction: Reducing agents such as sodium borohydride and lithium aluminum hydride are used.
Substitution: Nucleophiles like thiols and amines can be used under basic conditions.
Major Products Formed
The major products formed from these reactions include various quinones, dihydro derivatives, and substituted flavonoids .
Scientific Research Applications
Sophoraflavanone B has a wide range of scientific research applications:
Chemistry: It is used as a precursor for synthesizing other flavonoids and as a reagent in organic synthesis.
Biology: It is studied for its effects on cell signaling pathways and gene expression.
Medicine: It has potential therapeutic applications in hormone replacement therapy, cancer treatment, and as an antimicrobial agent.
Industry: It is used in the development of pharmaceuticals and nutraceuticals.
Mechanism of Action
Sophoraflavanone B exerts its effects primarily through its estrogenic activity. It binds to estrogen receptors ERα and ERβ, acting as a full agonist of ERα. This binding activates the estrogen receptor signaling pathways, leading to various biological effects, such as cell proliferation and gene expression. Additionally, it has been shown to inhibit enzymes like aromatase, which is involved in estrogen biosynthesis .
Comparison with Similar Compounds
Similar Compounds
8-Prenylnaringenin: Another name for sophoraflavanone B, known for its strong estrogenic activity.
Sophoraflavanone G: A related compound with similar estrogenic properties.
Kurarinone: Another prenylated flavonoid with estrogenic activity.
Uniqueness
Sophoraflavanone B is unique due to its strong estrogenic activity, which is higher than many other phytoestrogens. Its ability to act as a full agonist of ERα makes it particularly interesting for hormone replacement therapy and cancer research .
Biological Activity
Trimethyl arsenate (TMA), a methylated derivative of arsenic, is an organoarsenic compound with significant biological implications. Understanding its biological activity is crucial due to its potential toxicity and varying effects on human health and the environment. This article explores the biological activity of this compound, including its metabolism, toxicity, and potential therapeutic roles, supported by data tables and case studies.
This compound has the chemical formula and is classified as a methylated arsenic compound. It is produced through microbial methylation processes and can exist in various oxidation states, influencing its biological interactions.
Metabolism of this compound
This compound is primarily formed through the methylation of inorganic arsenic species. The methylation process involves the transfer of methyl groups from S-adenosylmethionine (AdoMet) to inorganic arsenic compounds, particularly As(III). The enzyme responsible for this transformation is ArsM, which catalyzes the formation of trimethylated species from trivalent arsenicals .
Methylation Pathway
The metabolic pathway can be summarized as follows:
- Inorganic Arsenic (As(III)) → Methylated intermediates (MMA(III), DMA(III)) → This compound (TMA)
- Enzymatic Reaction :
Toxicity Profile
The toxicity of this compound is significantly lower than that of inorganic arsenic compounds. However, it can still exhibit toxic effects under certain conditions:
- Acute Toxicity : TMA can be more toxic than inorganic arsenic in specific cellular contexts due to its ability to disrupt cellular processes.
- Chronic Exposure : Long-term exposure to this compound has been associated with various health issues, including skin lesions and peripheral neuropathy, similar to other arsenic compounds .
Comparative Toxicity of Arsenicals
The following table summarizes the relative toxicity of different arsenic species:
Compound | Toxicity Rank |
---|---|
MMA(III) | Most Toxic |
DMA(III) | Highly Toxic |
As(III) | Moderate Toxicity |
TMA | Lower Toxicity |
DMA(V), MMA(V) | Least Toxic |
Case Study 1: Chronic Exposure in Mexico
A study conducted in an arsenic-endemic region of Mexico examined urinary profiles of methylated arsenicals among residents exposed to high levels of inorganic arsenic. The findings indicated that urinary levels of trivalent methylated species (MAs III) were significantly higher in individuals with skin lesions compared to those without, suggesting a correlation between TMA exposure and increased susceptibility to arsenic toxicity .
Case Study 2: Microbial Methylation and Environmental Impact
Research involving Rhodopseudomonas palustris demonstrated that microbial action can convert inorganic arsenic into less toxic forms through methylation processes. This conversion not only reduces the bioavailability of toxic arsenic but also contributes to global arsenic cycling by producing volatile trimethylarsine gas, which escapes into the atmosphere, further mitigating environmental contamination .
Enzyme Interactions
This compound interacts with various enzymes within biological systems:
Properties
IUPAC Name |
trimethyl arsorate | |
---|---|---|
Source | PubChem | |
URL | https://pubchem.ncbi.nlm.nih.gov | |
Description | Data deposited in or computed by PubChem | |
InChI |
InChI=1S/C3H9AsO4/c1-6-4(5,7-2)8-3/h1-3H3 | |
Source | PubChem | |
URL | https://pubchem.ncbi.nlm.nih.gov | |
Description | Data deposited in or computed by PubChem | |
InChI Key |
IUOAVUNOSJBFAU-UHFFFAOYSA-N | |
Source | PubChem | |
URL | https://pubchem.ncbi.nlm.nih.gov | |
Description | Data deposited in or computed by PubChem | |
Canonical SMILES |
CO[As](=O)(OC)OC | |
Source | PubChem | |
URL | https://pubchem.ncbi.nlm.nih.gov | |
Description | Data deposited in or computed by PubChem | |
Molecular Formula |
C3H9AsO4 | |
Source | PubChem | |
URL | https://pubchem.ncbi.nlm.nih.gov | |
Description | Data deposited in or computed by PubChem | |
DSSTOX Substance ID |
DTXSID401047780 | |
Record name | Trimethyl arsenate | |
Source | EPA DSSTox | |
URL | https://comptox.epa.gov/dashboard/DTXSID401047780 | |
Description | DSSTox provides a high quality public chemistry resource for supporting improved predictive toxicology. | |
Molecular Weight |
184.02 g/mol | |
Source | PubChem | |
URL | https://pubchem.ncbi.nlm.nih.gov | |
Description | Data deposited in or computed by PubChem | |
CAS No. |
13006-30-9 | |
Record name | Trimethyl arsenate | |
Source | EPA DSSTox | |
URL | https://comptox.epa.gov/dashboard/DTXSID401047780 | |
Description | DSSTox provides a high quality public chemistry resource for supporting improved predictive toxicology. | |
Retrosynthesis Analysis
AI-Powered Synthesis Planning: Our tool employs the Template_relevance Pistachio, Template_relevance Bkms_metabolic, Template_relevance Pistachio_ringbreaker, Template_relevance Reaxys, Template_relevance Reaxys_biocatalysis model, leveraging a vast database of chemical reactions to predict feasible synthetic routes.
One-Step Synthesis Focus: Specifically designed for one-step synthesis, it provides concise and direct routes for your target compounds, streamlining the synthesis process.
Accurate Predictions: Utilizing the extensive PISTACHIO, BKMS_METABOLIC, PISTACHIO_RINGBREAKER, REAXYS, REAXYS_BIOCATALYSIS database, our tool offers high-accuracy predictions, reflecting the latest in chemical research and data.
Strategy Settings
Precursor scoring | Relevance Heuristic |
---|---|
Min. plausibility | 0.01 |
Model | Template_relevance |
Template Set | Pistachio/Bkms_metabolic/Pistachio_ringbreaker/Reaxys/Reaxys_biocatalysis |
Top-N result to add to graph | 6 |
Feasible Synthetic Routes
Disclaimer and Information on In-Vitro Research Products
Please be aware that all articles and product information presented on BenchChem are intended solely for informational purposes. The products available for purchase on BenchChem are specifically designed for in-vitro studies, which are conducted outside of living organisms. In-vitro studies, derived from the Latin term "in glass," involve experiments performed in controlled laboratory settings using cells or tissues. It is important to note that these products are not categorized as medicines or drugs, and they have not received approval from the FDA for the prevention, treatment, or cure of any medical condition, ailment, or disease. We must emphasize that any form of bodily introduction of these products into humans or animals is strictly prohibited by law. It is essential to adhere to these guidelines to ensure compliance with legal and ethical standards in research and experimentation.