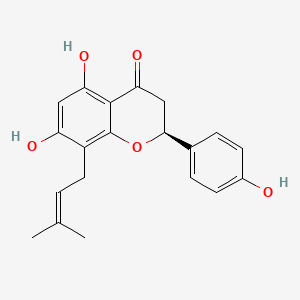
8-Prenylnaringenin
Overview
Description
8-Prenylnaringenin is a prenylflavonoid phytoestrogen, known for its potent estrogenic activity. It is found in hops (Humulus lupulus) and beer, and is considered one of the most estrogenic phytoestrogens known . The compound is equipotent at the two forms of estrogen receptors, ERα and ERβ, and acts as a full agonist of ERα .
Mechanism of Action
Target of Action
8-Prenylnaringenin (8-PN) is a prenylated flavonoid that occurs in hops and other plants . It is known to be one of the most potent phytoestrogens . The primary targets of 8-PN are the two forms of estrogen receptors, ERα and ERβ . These receptors play a crucial role in various biological systems, including the reproductive system, cardiovascular system, and skeletal system .
Mode of Action
8-PN interacts with its targets, the estrogen receptors, by binding to them . It acts as a full agonist of ERα . This means that it can bind to the receptor and activate it, leading to a biological response. The 8- substituent in 8-PN induces conformational changes in the receptor by binding of ligands, which impacts transcriptional outcomes .
Biochemical Pathways
The biochemical pathways affected by 8-PN are primarily related to estrogenic activity. As a phytoestrogen, 8-PN mimics the effects of the hormone estrogen in the body . It can induce the secretion of prolactin and increase other estrogenic responses . It also suppresses luteinizing hormone (LH) and follicle-stimulating hormone (FSH), indicating that it possesses antigonadotropic properties .
Result of Action
The molecular and cellular effects of 8-PN’s action are diverse. It has been shown to preserve bone density , reduce hot flashes , and activate proliferation of mammary cells . It also induces the expression of the progesterone receptor in various tissues . In an in vitro study, 8-PN and synthetic derivatives demonstrated anticancer properties .
Action Environment
The action, efficacy, and stability of 8-PN can be influenced by various environmental factors. For instance, the concentration of 8-PN found in beer is unlikely to have an estrogenic effect in breast tissue . Furthermore, the bioavailability of 8-PN is reportedly improved by prenylation . .
Biochemical Analysis
Biochemical Properties
8-PN interacts with various biomolecules, primarily the estrogen receptors ERα and ERβ . It acts as a full agonist of ERα, exhibiting effects similar to those of estradiol, albeit less potent .
Cellular Effects
8-PN has been shown to have various effects on cells. For instance, it has been demonstrated to reduce hot flashes , preserve bone density , and induce the secretion of prolactin . It also increases other estrogenic responses . In an in vivo study, 8-PN activated the proliferation of mammary cells .
Molecular Mechanism
The molecular mechanism of 8-PN involves its interaction with estrogen receptors ERα and ERβ . It acts as a full agonist of ERα . Its effects are similar to those of estradiol, but it is considerably less potent in comparison .
Temporal Effects in Laboratory Settings
It has been shown that 8-PN has various effects on cells and tissues, including the induction of the secretion of prolactin and the activation of the proliferation of mammary cells .
Dosage Effects in Animal Models
In animal models, the effects of 8-PN have been observed to vary with different dosages . For instance, it has been shown that both low and high doses of 8-PN can suppress serum LH and FSH, stimulate serum prolactin levels, and increase uterine weight .
Metabolic Pathways
The de novo biosynthetic pathway of 8-PN is composed of three parts: (1) (2 S)-naringenin synthesis; (2) dimethyllallyl diphosphate (DMAPP) synthesis through the mevalonate (MVA) pathway; and (3) prenylation of (2 S)-naringenin by PTs .
Transport and Distribution
It has been found that the amount of 8-PN in various tissues such as the kidneys, liver, muscles, lungs, pancreas, heart, spleen, and brain was higher than that of nonprenylated naringenin .
Subcellular Localization
It has been found that the gene expression of SfN8DT-1, which is responsible for the prenylation of the flavonoid naringenin at the 8-position, is strictly limited to the root bark where prenylated flavonoids are solely accumulated in planta .
Preparation Methods
Synthetic Routes and Reaction Conditions: 8-Prenylnaringenin can be synthesized through various methods. One approach involves the use of prenyltransferases (PTs) from different sources. For instance, the enzyme Sf N8DT-1 derived from Sophora flavescens can synthesize this compound . The process involves multiple sequence alignment and mutagenesis to enhance catalytic activity. Overexpression of key genes in the mevalonate pathway can significantly improve the yield of this compound .
Industrial Production Methods: Industrial production of this compound often involves microbial biosynthesis. Saccharomyces cerevisiae, a type of yeast, can be engineered to produce this compound by enhancing precursor pathways and optimizing enzyme activity . This method has shown promising results, with significant improvements in yield through metabolic and protein engineering .
Chemical Reactions Analysis
Types of Reactions: 8-Prenylnaringenin undergoes various chemical reactions, including oxidation, reduction, and substitution. These reactions are influenced by the presence of hydroxyl groups and the prenyl side chain.
Common Reagents and Conditions:
Oxidation: Common oxidizing agents such as potassium permanganate (KMnO₄) and hydrogen peroxide (H₂O₂) can be used to oxidize this compound.
Reduction: Reducing agents like sodium borohydride (NaBH₄) are employed to reduce the compound.
Substitution: Substitution reactions often involve nucleophiles such as halides and amines.
Major Products Formed: The major products formed from these reactions include various hydroxylated and prenylated derivatives, which can exhibit different biological activities.
Scientific Research Applications
8-Prenylnaringenin has a wide range of scientific research applications:
Chemistry: It is used as a precursor for synthesizing other prenylated flavonoids.
Biology: The compound is studied for its estrogenic effects and potential role in hormone replacement therapy.
Medicine: Research has shown that this compound can reduce hot flashes, preserve bone density, and exhibit anticancer properties
Industry: It is used in the brewing industry to enhance the estrogenic effects of beer.
Comparison with Similar Compounds
Naringenin: A flavanone found in citrus fruits, known for its antioxidant and anti-inflammatory properties.
Isoxanthohumol: A prenylflavonoid found in hops, with lower estrogenic activity compared to 8-Prenylnaringenin.
Xanthohumol: Another prenylflavonoid from hops, studied for its anticancer and anti-inflammatory effects.
Uniqueness of this compound: this compound stands out due to its potent estrogenic activity, making it a valuable compound for studying hormone replacement therapy and other estrogen-related effects . Its ability to act as a full agonist of ERα and its significant impact on various biological pathways highlight its uniqueness among similar compounds .
Properties
IUPAC Name |
(2S)-5,7-dihydroxy-2-(4-hydroxyphenyl)-8-(3-methylbut-2-enyl)-2,3-dihydrochromen-4-one | |
---|---|---|
Source | PubChem | |
URL | https://pubchem.ncbi.nlm.nih.gov | |
Description | Data deposited in or computed by PubChem | |
InChI |
InChI=1S/C20H20O5/c1-11(2)3-8-14-15(22)9-16(23)19-17(24)10-18(25-20(14)19)12-4-6-13(21)7-5-12/h3-7,9,18,21-23H,8,10H2,1-2H3/t18-/m0/s1 | |
Source | PubChem | |
URL | https://pubchem.ncbi.nlm.nih.gov | |
Description | Data deposited in or computed by PubChem | |
InChI Key |
LPEPZZAVFJPLNZ-SFHVURJKSA-N | |
Source | PubChem | |
URL | https://pubchem.ncbi.nlm.nih.gov | |
Description | Data deposited in or computed by PubChem | |
Canonical SMILES |
CC(=CCC1=C2C(=C(C=C1O)O)C(=O)CC(O2)C3=CC=C(C=C3)O)C | |
Source | PubChem | |
URL | https://pubchem.ncbi.nlm.nih.gov | |
Description | Data deposited in or computed by PubChem | |
Isomeric SMILES |
CC(=CCC1=C2C(=C(C=C1O)O)C(=O)C[C@H](O2)C3=CC=C(C=C3)O)C | |
Source | PubChem | |
URL | https://pubchem.ncbi.nlm.nih.gov | |
Description | Data deposited in or computed by PubChem | |
Molecular Formula |
C20H20O5 | |
Source | PubChem | |
URL | https://pubchem.ncbi.nlm.nih.gov | |
Description | Data deposited in or computed by PubChem | |
DSSTOX Substance ID |
DTXSID60333083 | |
Record name | 8-Prenylnaringenin | |
Source | EPA DSSTox | |
URL | https://comptox.epa.gov/dashboard/DTXSID60333083 | |
Description | DSSTox provides a high quality public chemistry resource for supporting improved predictive toxicology. | |
Molecular Weight |
340.4 g/mol | |
Source | PubChem | |
URL | https://pubchem.ncbi.nlm.nih.gov | |
Description | Data deposited in or computed by PubChem | |
CAS No. |
53846-50-7 | |
Record name | (-)-8-Prenylnaringenin | |
Source | CAS Common Chemistry | |
URL | https://commonchemistry.cas.org/detail?cas_rn=53846-50-7 | |
Description | CAS Common Chemistry is an open community resource for accessing chemical information. Nearly 500,000 chemical substances from CAS REGISTRY cover areas of community interest, including common and frequently regulated chemicals, and those relevant to high school and undergraduate chemistry classes. This chemical information, curated by our expert scientists, is provided in alignment with our mission as a division of the American Chemical Society. | |
Explanation | The data from CAS Common Chemistry is provided under a CC-BY-NC 4.0 license, unless otherwise stated. | |
Record name | 8-Prenylnaringenin | |
Source | ChemIDplus | |
URL | https://pubchem.ncbi.nlm.nih.gov/substance/?source=chemidplus&sourceid=0053846507 | |
Description | ChemIDplus is a free, web search system that provides access to the structure and nomenclature authority files used for the identification of chemical substances cited in National Library of Medicine (NLM) databases, including the TOXNET system. | |
Record name | 8-Prenylnaringenin | |
Source | EPA DSSTox | |
URL | https://comptox.epa.gov/dashboard/DTXSID60333083 | |
Description | DSSTox provides a high quality public chemistry resource for supporting improved predictive toxicology. | |
Record name | 8-PRENYLNARINGENIN | |
Source | FDA Global Substance Registration System (GSRS) | |
URL | https://gsrs.ncats.nih.gov/ginas/app/beta/substances/5L872SZR8X | |
Description | The FDA Global Substance Registration System (GSRS) enables the efficient and accurate exchange of information on what substances are in regulated products. Instead of relying on names, which vary across regulatory domains, countries, and regions, the GSRS knowledge base makes it possible for substances to be defined by standardized, scientific descriptions. | |
Explanation | Unless otherwise noted, the contents of the FDA website (www.fda.gov), both text and graphics, are not copyrighted. They are in the public domain and may be republished, reprinted and otherwise used freely by anyone without the need to obtain permission from FDA. Credit to the U.S. Food and Drug Administration as the source is appreciated but not required. | |
Retrosynthesis Analysis
AI-Powered Synthesis Planning: Our tool employs the Template_relevance Pistachio, Template_relevance Bkms_metabolic, Template_relevance Pistachio_ringbreaker, Template_relevance Reaxys, Template_relevance Reaxys_biocatalysis model, leveraging a vast database of chemical reactions to predict feasible synthetic routes.
One-Step Synthesis Focus: Specifically designed for one-step synthesis, it provides concise and direct routes for your target compounds, streamlining the synthesis process.
Accurate Predictions: Utilizing the extensive PISTACHIO, BKMS_METABOLIC, PISTACHIO_RINGBREAKER, REAXYS, REAXYS_BIOCATALYSIS database, our tool offers high-accuracy predictions, reflecting the latest in chemical research and data.
Strategy Settings
Precursor scoring | Relevance Heuristic |
---|---|
Min. plausibility | 0.01 |
Model | Template_relevance |
Template Set | Pistachio/Bkms_metabolic/Pistachio_ringbreaker/Reaxys/Reaxys_biocatalysis |
Top-N result to add to graph | 6 |
Feasible Synthetic Routes
Q1: What is 8-prenylnaringenin and where is it found?
A1: this compound (8-PN) is a prenylated flavonoid primarily found in the female flowers of the hop plant (Humulus lupulus L.). It's a natural constituent of beer, though in low concentrations, and is also being investigated for its potential use in dietary supplements. [, ]
Q2: this compound is described as a phytoestrogen. What does this mean?
A2: Phytoestrogens are plant-derived compounds that can exert estrogen-like effects in the body. 8-PN is considered one of the most potent phytoestrogens identified, exhibiting higher estrogenic activity in vitro than other known phytoestrogens like coumestrol, genistein, and daidzein. [, ]
Q3: How does this compound exert its estrogenic effects?
A3: 8-PN exerts its effects by binding to estrogen receptors (ERs), specifically showing a preference for ERα over ERβ. [, ] This binding interaction triggers downstream signaling pathways, leading to the regulation of gene expression and various physiological responses in estrogen-responsive tissues. [, ]
Q4: How does the estrogenic potency of this compound compare to other hop flavonoids?
A4: this compound significantly surpasses the estrogenic potency of other hop flavonoids, including 6-prenylnaringenin, 6,8-diprenylnaringenin, and 8-geranylnaringenin. While these compounds display some estrogenic activity, their potency is less than 1% of that exhibited by 8-PN. []
Q5: this compound is said to influence the E-cadherin/catenin complex. What is the significance of this interaction?
A6: The E-cadherin/catenin complex plays a crucial role in cell-to-cell adhesion and acts as an invasion suppressor in epithelial cells. 8-PN has been shown to stimulate E-cadherin-dependent aggregation and growth in MCF-7/6 breast cancer cells, mimicking the effects of 17β-estradiol. This suggests a potential role for 8-PN in inhibiting tumor invasion and metastasis. []
Q6: Is isoxanthohumol related to this compound?
A7: Yes, isoxanthohumol (IX) is a prenylflavonoid found in hops and beer that acts as a prodrug to 8-PN. This means IX itself is not estrogenic, but can be converted into the potent phytoestrogen 8-PN within the body. []
Q7: How is isoxanthohumol converted into this compound?
A8: The conversion of IX into 8-PN primarily occurs through O-demethylation by the intestinal microbiota, specifically by bacteria residing in the distal colon. [, ] This microbial biotransformation process highlights the crucial role of the gut microbiome in determining the final biological activity of ingested hop compounds.
Q8: Are there individual differences in the conversion of isoxanthohumol to this compound?
A9: Yes, there's significant interindividual variability in the ability of the gut microbiota to convert IX into 8-PN. Studies have shown that approximately one-third of individuals are efficient converters (Hop+), while the remaining exhibit low conversion rates (Hop-). This variability is attributed to differences in the composition of their intestinal microbiota. []
Q9: Can the conversion of isoxanthohumol to this compound be enhanced?
A10: Research suggests that administration of specific bacterial strains, such as Eubacterium limosum, can enhance the conversion of IX into 8-PN, even in individuals with low conversion capabilities (Hop-). This probiotic approach holds potential for balancing phytoestrogen exposure from hop consumption. []
Q10: What are the primary metabolic pathways of this compound in humans?
A11: In humans, 8-PN is primarily metabolized in the liver. The main metabolic reactions involve: * Oxidation of the prenyl side chain, primarily at the terminal methyl groups, leading to the formation of trans- and cis-alcohols.* Oxidation of the flavanone skeleton, mainly at the 3' position on the B ring.* Epoxide formation on the prenyl group double bond, followed by intramolecular cyclization.* Desaturation of the C ring, resulting in the formation of 8-prenylapigenin.* Ipso hydroxylation of the B ring, potentially leading to B ring cleavage and the formation of 8-prenylchromone. []
Q11: Which cytochrome P450 enzymes are involved in the metabolism of isoxanthohumol and this compound?
A11: Specific cytochrome P450 (CYP) enzymes play key roles in metabolizing IX and 8-PN. These include:
Q12: What are the potential therapeutic benefits of this compound?
A12: Given its potent estrogenic activity, 8-PN has garnered interest for its potential in addressing conditions associated with estrogen deficiency, primarily menopausal symptoms. Research suggests it could offer benefits in:
- Alleviating Menopausal Symptoms: Clinical trials indicate that hop-containing preparations, likely due to 8-PN, can significantly reduce the frequency of hot flashes, a common and bothersome symptom of menopause. []
- Preventing Bone Loss: 8-PN's estrogenic effects suggest potential in mitigating bone loss associated with estrogen deficiency, a crucial factor in osteoporosis development. []
- Inhibiting Tumor Growth: In vitro studies highlight the ability of 8-PN and related prenylflavanones to induce apoptosis (programmed cell death) in cancer cells, suggesting potential as anticancer agents. [, ]
Q13: What effects does this compound have on bone cells?
A15: In vitro studies show that 8-PN promotes the differentiation and mineralization of osteoblasts, the cells responsible for bone formation. [, ] Additionally, it inhibits the formation and activity of osteoclasts, the cells that break down bone. [] These findings suggest that 8-PN might help maintain bone density and prevent bone loss.
Q14: How does this compound affect muscle recovery from disuse atrophy?
A16: 8-PN has shown potential in promoting recovery from muscle atrophy caused by disuse, such as that experienced during immobilization. Studies suggest it achieves this by activating the PI3K/Akt/P70S6K1 pathway, a crucial signaling cascade for muscle protein synthesis and growth. []
Q15: Could this compound be a potential treatment for neurodegenerative diseases?
A17: While research is in its early stages, 8-PN and related compounds have shown potential in promoting the differentiation of neural precursor cells into neurons. [] This neurodifferentiating potential, along with its ability to cross the blood-brain barrier, makes it a molecule of interest for exploring potential therapeutic applications in neurodegenerative diseases.
Q16: Are there any safety concerns associated with the consumption of this compound?
A16: While 8-PN holds promise for various therapeutic applications, it's crucial to acknowledge potential safety concerns:
- Estrogenic Effects: Given its potent estrogenic activity, long-term exposure to high doses of 8-PN might lead to adverse effects in estrogen-sensitive tissues. More research is needed to determine safe and effective dosages for long-term use. []
Q17: What further research is needed to fully understand the therapeutic potential of this compound?
A17: Further research is needed to:
Disclaimer and Information on In-Vitro Research Products
Please be aware that all articles and product information presented on BenchChem are intended solely for informational purposes. The products available for purchase on BenchChem are specifically designed for in-vitro studies, which are conducted outside of living organisms. In-vitro studies, derived from the Latin term "in glass," involve experiments performed in controlled laboratory settings using cells or tissues. It is important to note that these products are not categorized as medicines or drugs, and they have not received approval from the FDA for the prevention, treatment, or cure of any medical condition, ailment, or disease. We must emphasize that any form of bodily introduction of these products into humans or animals is strictly prohibited by law. It is essential to adhere to these guidelines to ensure compliance with legal and ethical standards in research and experimentation.