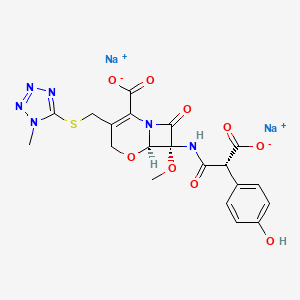
Moxalactam disodium
Overview
Description
Moxalactam Disodium: is a semisynthetic beta-lactam antibiotic belonging to the oxacephem class. It is known for its broad-spectrum antibacterial properties and is effective against both Gram-positive and Gram-negative bacteria. This compound is particularly used to treat respiratory tract infections, urinary tract infections, intra-abdominal infections, and septicemia .
Mechanism of Action
- Moxalactam disodium, also known as latamoxef , belongs to the oxacephem class of antibiotics. It is structurally related to cephalosporins but has an oxygen atom instead of a sulfur atom in its core structure .
- PBPs play a crucial role in bacterial cell wall synthesis. By acylating the PBP, moxalactam inhibits the formation of cross-links between linear peptidoglycan strands, thereby disrupting the final stage of bacterial cell wall assembly .
- This inactivation of the enzyme prevents the formation of cross-links between peptidoglycan strands, ultimately inhibiting cell wall synthesis .
Target of Action
Mode of Action
Result of Action
Biochemical Analysis
Biochemical Properties
Moxalactam disodium plays a crucial role in inhibiting bacterial cell wall synthesis. It specifically targets penicillin-binding proteins (PBPs), which are essential enzymes that catalyze the cross-linking of the peptidoglycan layer, a critical component of the bacterial cell wall. By binding to these PBPs, this compound disrupts the final stages of cell wall assembly, leading to the formation of weak and unstable cell walls . This disruption results in osmotic instability, causing bacterial cells to swell and eventually burst due to the inability to maintain cellular integrity .
Cellular Effects
This compound exerts significant effects on various types of cells and cellular processes. It influences cell function by inhibiting cell wall synthesis, which is vital for bacterial survival. This inhibition leads to cell lysis and death in susceptible bacterial strains. Additionally, this compound has been shown to affect cell signaling pathways, gene expression, and cellular metabolism by disrupting the integrity of the bacterial cell wall . The compound’s broad-spectrum activity makes it effective against a variety of pathogens, including those responsible for respiratory tract infections, urinary tract infections, intra-abdominal infections, and septicemia .
Molecular Mechanism
At the molecular level, this compound exerts its effects by binding to penicillin-binding proteins (PBPs) and inhibiting their activity. This binding prevents the cross-linking of peptidoglycan chains, which is essential for maintaining the structural integrity of the bacterial cell wall . The inhibition of PBPs leads to the accumulation of peptidoglycan precursors, resulting in cell wall weakening and eventual cell lysis. This compound is also resistant to hydrolysis by beta-lactamases, enzymes produced by some bacteria to inactivate beta-lactam antibiotics .
Temporal Effects in Laboratory Settings
In laboratory settings, the effects of this compound have been observed to change over time. The compound is stable under various conditions, but its efficacy can be influenced by factors such as pH, temperature, and the presence of other substances. Studies have shown that this compound maintains its antibacterial activity over extended periods, with minimal degradation . Long-term effects on cellular function have been observed in both in vitro and in vivo studies, indicating sustained antibacterial activity and low toxicity .
Dosage Effects in Animal Models
The effects of this compound vary with different dosages in animal models. Studies have demonstrated that higher doses of the compound result in increased antibacterial activity, but also a higher incidence of adverse effects. In rats, dogs, and monkeys, dosages ranging from 100 to 3,500 mg/kg per day have been studied, with treatment-related effects including soft stool, cecal dilatation, and slight anemia at higher dosages . These effects were reversible and less severe than those caused by other beta-lactam antibiotics .
Metabolic Pathways
This compound is primarily excreted unchanged through the kidneys, with minimal metabolism occurring in the body . The compound is eliminated via glomerular filtration, and its serum half-life is prolonged in individuals with impaired renal function . No active metabolites of this compound have been detected in plasma or urine, indicating that the parent compound is responsible for its antibacterial activity .
Transport and Distribution
This compound is transported and distributed within cells and tissues primarily through the bloodstream. After intravenous administration, the compound rapidly reaches therapeutic levels in the serum and is distributed to various tissues, including bile and sputum . The compound’s distribution is influenced by its protein binding capacity, which ranges from 35% to 50% . This compound is also capable of penetrating into infected sites, such as subcutaneous abscesses, where it maintains its antibacterial activity .
Subcellular Localization
The subcellular localization of this compound is primarily within the bacterial cell wall, where it exerts its antibacterial effects. The compound targets penicillin-binding proteins located in the cell membrane, leading to the inhibition of cell wall synthesis . This compound does not undergo significant post-translational modifications or targeting signals, as its primary mode of action is through direct interaction with bacterial cell wall components .
Preparation Methods
Synthetic Routes and Reaction Conditions: Moxalactam Disodium is synthesized through a series of chemical reactions starting from 6-aminopenicillanic acid. The process involves several steps, including chlorination, displacement with propargyl alcohol, partial reduction, epoxidation, and opening of the epoxide ring. The final stages involve oxidation, ozonolysis, and intramolecular Wittig reaction .
Industrial Production Methods: Industrial production of this compound involves large-scale synthesis using the same chemical routes as in laboratory synthesis but optimized for higher yields and purity. The process includes stringent quality control measures to ensure the final product meets pharmaceutical standards .
Chemical Reactions Analysis
Types of Reactions: Moxalactam Disodium undergoes various chemical reactions, including:
Oxidation: Involves the addition of oxygen or the removal of hydrogen.
Reduction: Involves the addition of hydrogen or the removal of oxygen.
Substitution: Involves the replacement of one functional group with another.
Common Reagents and Conditions:
Oxidation: Common reagents include potassium permanganate and hydrogen peroxide.
Reduction: Common reagents include sodium borohydride and lithium aluminum hydride.
Substitution: Common reagents include halogens and nucleophiles under basic or acidic conditions.
Major Products Formed: The major products formed from these reactions depend on the specific conditions and reagents used. For example, oxidation can lead to the formation of carboxylic acids, while reduction can produce alcohols .
Scientific Research Applications
Moxalactam Disodium has a wide range of scientific research applications:
Chemistry: Used as a model compound to study beta-lactam antibiotics and their interactions with bacterial enzymes.
Biology: Investigated for its effects on bacterial cell wall synthesis and resistance mechanisms.
Medicine: Used in clinical trials to evaluate its efficacy and safety in treating various bacterial infections.
Industry: Employed in the development of new antibiotics and as a standard for quality control in pharmaceutical manufacturing
Comparison with Similar Compounds
Cefotaxime: Another beta-lactam antibiotic with a similar mechanism of action but different spectrum of activity.
Cefoperazone: Known for its activity against Pseudomonas aeruginosa, which Moxalactam Disodium has moderate activity against.
Ceftriaxone: A third-generation cephalosporin with a longer half-life compared to this compound
Uniqueness: this compound is unique due to its structural modification where the sulfur atom in the cephalosporin core is replaced with an oxygen atom. This substitution provides greater antibacterial activity and stability against beta-lactamases compared to its cephalosporin analogs .
Properties
CAS No. |
64953-12-4 |
---|---|
Molecular Formula |
C20H18N6Na2O9S |
Molecular Weight |
564.4 g/mol |
IUPAC Name |
disodium;(7R)-7-[[2-carboxylato-2-(4-hydroxyphenyl)acetyl]amino]-7-methoxy-3-[(1-methyltetrazol-5-yl)sulfanylmethyl]-8-oxo-5-oxa-1-azabicyclo[4.2.0]oct-2-ene-2-carboxylate |
InChI |
InChI=1S/C20H20N6O9S.2Na/c1-25-19(22-23-24-25)36-8-10-7-35-18-20(34-2,17(33)26(18)13(10)16(31)32)21-14(28)12(15(29)30)9-3-5-11(27)6-4-9;;/h3-6,12,18,27H,7-8H2,1-2H3,(H,21,28)(H,29,30)(H,31,32);;/q;2*+1/p-2/t12?,18?,20-;;/m0../s1 |
InChI Key |
GRIXGZQULWMCLU-PJDRSYKOSA-L |
Isomeric SMILES |
CN1C(=NN=N1)SCC2=C(N3C([C@@](C3=O)(NC(=O)C(C4=CC=C(C=C4)O)C(=O)[O-])OC)OC2)C(=O)[O-].[Na+].[Na+] |
SMILES |
CN1C(=NN=N1)SCC2=C(N3C(C(C3=O)(NC(=O)C(C4=CC=C(C=C4)O)C(=O)[O-])OC)OC2)C(=O)[O-].[Na+].[Na+] |
Canonical SMILES |
CN1C(=NN=N1)SCC2=C(N3C(C(C3=O)(NC(=O)C(C4=CC=C(C=C4)O)C(=O)[O-])OC)OC2)C(=O)[O-].[Na+].[Na+] |
Appearance |
Solid powder |
64953-12-4 | |
Pictograms |
Irritant; Health Hazard |
Purity |
>98% (or refer to the Certificate of Analysis) |
shelf_life |
>2 years if stored properly |
solubility |
Soluble in DMSO |
storage |
Dry, dark and at 0 - 4 C for short term (days to weeks) or -20 C for long term (months to years). |
Synonyms |
1 Oxacephalosporin 1-Oxacephalosporin 6059 S 6059-S 6059S Disodium Latamoxef Disodium Moxalactam Disodium, Moxalactam Festamoxin Lamoxactam Latamoxef Latamoxef, Disodium Lilly 127935 Ly 127935 Ly-127935 Ly127935 Moxalactam Moxalactam Disodium Moxalactam, Disodium S 6059 S-6059 S6059 Shiomarin |
Origin of Product |
United States |
Retrosynthesis Analysis
AI-Powered Synthesis Planning: Our tool employs the Template_relevance Pistachio, Template_relevance Bkms_metabolic, Template_relevance Pistachio_ringbreaker, Template_relevance Reaxys, Template_relevance Reaxys_biocatalysis model, leveraging a vast database of chemical reactions to predict feasible synthetic routes.
One-Step Synthesis Focus: Specifically designed for one-step synthesis, it provides concise and direct routes for your target compounds, streamlining the synthesis process.
Accurate Predictions: Utilizing the extensive PISTACHIO, BKMS_METABOLIC, PISTACHIO_RINGBREAKER, REAXYS, REAXYS_BIOCATALYSIS database, our tool offers high-accuracy predictions, reflecting the latest in chemical research and data.
Strategy Settings
Precursor scoring | Relevance Heuristic |
---|---|
Min. plausibility | 0.01 |
Model | Template_relevance |
Template Set | Pistachio/Bkms_metabolic/Pistachio_ringbreaker/Reaxys/Reaxys_biocatalysis |
Top-N result to add to graph | 6 |
Feasible Synthetic Routes
Disclaimer and Information on In-Vitro Research Products
Please be aware that all articles and product information presented on BenchChem are intended solely for informational purposes. The products available for purchase on BenchChem are specifically designed for in-vitro studies, which are conducted outside of living organisms. In-vitro studies, derived from the Latin term "in glass," involve experiments performed in controlled laboratory settings using cells or tissues. It is important to note that these products are not categorized as medicines or drugs, and they have not received approval from the FDA for the prevention, treatment, or cure of any medical condition, ailment, or disease. We must emphasize that any form of bodily introduction of these products into humans or animals is strictly prohibited by law. It is essential to adhere to these guidelines to ensure compliance with legal and ethical standards in research and experimentation.