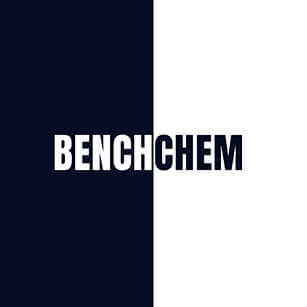
cefotaxime
- Click on QUICK INQUIRY to receive a quote from our team of experts.
- With the quality product at a COMPETITIVE price, you can focus more on your research.
Overview
Description
Cefotaxime is a third-generation cephalosporin antibiotic used to treat a variety of bacterial infections. It is effective against both Gram-positive and Gram-negative bacteria. This compound is commonly used to treat infections such as pneumonia, urinary tract infections, meningitis, and sepsis . It works by inhibiting bacterial cell wall synthesis, leading to the death of the bacteria .
Preparation Methods
Cefotaxime is synthesized through a series of chemical reactions. One common method involves the condensation of 7-aminocephalosporanic acid (7-ACA) with methoxyiminoacetic acid to form this compound acid. This intermediate is then converted to this compound sodium through a series of purification and crystallization steps . Industrial production methods often involve the use of solvents such as dichloromethane and ethyl alcohol, and reagents like triethylamine .
Chemical Reactions Analysis
Cefotaxime undergoes various chemical reactions, including:
Oxidation: this compound can be oxidized under certain conditions, leading to the formation of degradation products.
Reduction: Reduction reactions are less common but can occur under specific conditions.
Substitution: this compound can undergo substitution reactions, particularly in the presence of nucleophiles.
Hydrolysis: This is a significant reaction for this compound, especially in aqueous solutions, leading to the formation of desacetylthis compound
Common reagents used in these reactions include acids, bases, and various organic solvents. The major products formed from these reactions are often degradation products that can affect the stability and efficacy of the antibiotic .
Scientific Research Applications
Cefotaxime has a wide range of applications in scientific research:
Chemistry: It is used in the synthesis of various derivatives and analogs for studying structure-activity relationships.
Biology: this compound is used in plant tissue culture to eliminate bacterial contamination.
Medicine: It is extensively used in clinical settings to treat severe bacterial infections.
Industry: This compound-loaded nanoparticles are being researched for their potential in drug delivery systems .
Mechanism of Action
Cefotaxime exerts its effects by binding to penicillin-binding proteins (PBPs) in the bacterial cell wall. This binding inhibits the final transpeptidation step of peptidoglycan synthesis, which is essential for cell wall integrity. As a result, the bacterial cell wall weakens, leading to cell lysis and death .
Comparison with Similar Compounds
Cefotaxime is often compared with other third-generation cephalosporins such as ceftriaxone and ceftazidime. While all three antibiotics have broad-spectrum activity, this compound is unique in its high affinity for PBPs and its effectiveness against a wide range of bacteria. Ceftriaxone is known for its longer half-life, allowing for less frequent dosing, while ceftazidime is particularly effective against Pseudomonas aeruginosa .
Similar compounds include:
- Ceftriaxone
- Ceftazidime
- Cefuroxime
- Cefepime
Each of these antibiotics has its own unique properties and clinical applications, making them valuable tools in the treatment of bacterial infections .
Properties
CAS No. |
152186-43-1 |
---|---|
Molecular Formula |
2C33H36N2O4.C4H4O4 |
Molecular Weight |
0 |
Synonyms |
5-Thia-1-azabicyclo[4.2.0]oct-2-ene-2-carboxylic acid, 3-[(acetyloxy)methyl]-7-[[(2-amino-4-thiazolyl)(methoxyimino)acetyl]amino]-8-oxo-, [6R-[6α,7α(Z)]]- |
Origin of Product |
United States |
Disclaimer and Information on In-Vitro Research Products
Please be aware that all articles and product information presented on BenchChem are intended solely for informational purposes. The products available for purchase on BenchChem are specifically designed for in-vitro studies, which are conducted outside of living organisms. In-vitro studies, derived from the Latin term "in glass," involve experiments performed in controlled laboratory settings using cells or tissues. It is important to note that these products are not categorized as medicines or drugs, and they have not received approval from the FDA for the prevention, treatment, or cure of any medical condition, ailment, or disease. We must emphasize that any form of bodily introduction of these products into humans or animals is strictly prohibited by law. It is essential to adhere to these guidelines to ensure compliance with legal and ethical standards in research and experimentation.