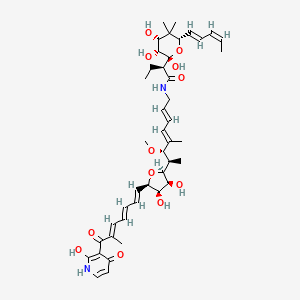
Kirromycin
- Click on QUICK INQUIRY to receive a quote from our team of experts.
- With the quality product at a COMPETITIVE price, you can focus more on your research.
Overview
Description
Mechanism of Action
Target of Action
Kirromycin, also known as Delvomycin or this compound, primarily targets the elongation factor Tu (EF-Tu) in prokaryotes . EF-Tu is a crucial component in the protein synthesis machinery of bacteria, playing a significant role in the elongation phase of protein synthesis .
Mode of Action
This compound interacts with EF-Tu, inhibiting the substrate supply during the elongation phase of protein synthesis . Unlike some other antibiotics, such as fusidic acid, this compound selectively acts upon the elongation factor from prokaryotes . This interaction maintains the EF-Tu*GTP conformation even after GTP is hydrolyzed to GDP .
Biochemical Pathways
The biosynthesis of this compound involves non-ribosomal peptide synthetases (NRPSs), acyltransferase (AT)-harbouring polyketide synthases (PKSs), and AT-less PKSs . These enzymes catalyze the formation of complex secondary metabolites such as this compound . The biosynthetic steps involve the formation of a phosphopantetheinyl group attached to a conserved serine residue within the active site .
Pharmacokinetics
It is known that the compound’s bioavailability and efficacy can be influenced by various factors, including its chemical structure and the presence of other compounds .
Result of Action
The result of this compound’s action is the inhibition of protein synthesis in bacteria. By acting on EF-Tu, this compound blocks the substrate supply necessary for the elongation phase of protein synthesis . This leads to the cessation of protein synthesis and ultimately inhibits bacterial growth .
Action Environment
The action, efficacy, and stability of this compound can be influenced by environmental factors. For instance, the incorporation of polystyrenic resins into the fermentation process of Actinoplanes sp A8924, a producer of this compound, resulted in a fourfold enhancement of this compound productivity . This suggests that the production and action of this compound can be optimized under certain environmental conditions .
Biochemical Analysis
Biochemical Properties
Kirromycin is synthesized via a unique combination of trans-/cis-AT type I polyketide synthases and non-ribosomal peptide synthetases (PKS I/NRPS) . The biosynthetic pathway involves several enzymes, including tailoring enzymes KirM, KirHVI, KirOI, and KirOII, and the putative crotonyl-CoA reductase/carboxylase KirN .
Cellular Effects
This compound exerts its effects by binding to the elongation factor (EF) Tu in prokaryotes . This interaction inhibits protein biosynthesis, impacting various cellular processes.
Molecular Mechanism
This compound’s mechanism of action involves binding interactions with biomolecules, specifically the elongation factor EF-Tu . This binding inhibits the function of EF-Tu, leading to the inhibition of protein biosynthesis .
Temporal Effects in Laboratory Settings
The effects of this compound have been studied over time in laboratory settings
Metabolic Pathways
This compound is involved in several metabolic pathways, interacting with enzymes and cofactors
Preparation Methods
Synthetic Routes and Reaction Conditions
The synthesis of mocimycin involves complex organic reactions. The antibiotic is typically isolated from the culture fluids of Streptomyces ramocissimus. The process includes fermentation, extraction, and purification steps. The fermentation is carried out under controlled conditions to optimize the yield of mocimycin. After fermentation, the compound is extracted using organic solvents and purified through chromatographic techniques .
Industrial Production Methods
Industrial production of mocimycin follows similar steps but on a larger scale. The fermentation process is scaled up in bioreactors, and the extraction and purification processes are optimized for efficiency and yield. The use of advanced biotechnological methods and equipment ensures the consistent production of high-purity mocimycin .
Chemical Reactions Analysis
Types of Reactions
Mocimycin undergoes various chemical reactions, including:
Oxidation: Mocimycin can be oxidized under specific conditions to form different derivatives.
Reduction: Reduction reactions can modify the functional groups in mocimycin, leading to the formation of reduced derivatives.
Substitution: Mocimycin can undergo substitution reactions where functional groups are replaced by other groups.
Common Reagents and Conditions
Common reagents used in these reactions include oxidizing agents like potassium permanganate, reducing agents like sodium borohydride, and various nucleophiles for substitution reactions. The reaction conditions, such as temperature, pH, and solvent, are carefully controlled to achieve the desired products .
Major Products Formed
The major products formed from these reactions include various derivatives of mocimycin with modified functional groups. These derivatives can have different biological activities and properties, making them useful for further research and applications .
Scientific Research Applications
Mocimycin has a wide range of scientific research applications:
Chemistry: Mocimycin is used as a model compound for studying antibiotic synthesis and mechanisms of action.
Biology: It is used to study bacterial protein synthesis and the role of elongation factors in this process.
Medicine: Mocimycin has potential therapeutic applications in treating bacterial infections, particularly those caused by antibiotic-resistant strains.
Comparison with Similar Compounds
Mocimycin is part of the elfamycin family of antibiotics, which includes other compounds such as:
- Pulvomycin
- Aurodox
- Heneicomycin
- Efrotomycin
These compounds share a similar mechanism of action but differ in their chemical structures and specific biological activities. Mocimycin is unique in its specific binding to EF-Tu and its effectiveness against certain bacterial strains .
Properties
CAS No. |
50935-71-2 |
---|---|
Molecular Formula |
C43H60N2O12 |
Molecular Weight |
796.9 g/mol |
IUPAC Name |
(2S)-N-[(2E,4E,6S,7R)-7-[(2S,3S,4R,5R)-3,4-dihydroxy-5-[(1E,3E,5E)-7-(4-hydroxy-2-oxo-1H-pyridin-3-yl)-6-methyl-7-oxohepta-1,3,5-trienyl]oxolan-2-yl]-6-methoxy-5-methylocta-2,4-dienyl]-2-[(2R,3R,4R,6S)-2,3,4-trihydroxy-5,5-dimethyl-6-[(1E,3E)-penta-1,3-dienyl]oxan-2-yl]butanamide |
InChI |
InChI=1S/C43H60N2O12/c1-9-11-13-21-31-42(6,7)38(50)39(51)43(54,57-31)28(10-2)40(52)44-23-17-16-19-26(4)36(55-8)27(5)37-35(49)34(48)30(56-37)20-15-12-14-18-25(3)33(47)32-29(46)22-24-45-41(32)53/h9,11-22,24,27-28,30-31,34-39,48-51,54H,10,23H2,1-8H3,(H,44,52)(H2,45,46,53)/b11-9+,14-12+,17-16+,20-15+,21-13+,25-18+,26-19+/t27-,28-,30-,31+,34+,35+,36-,37+,38+,39-,43-/m1/s1 |
InChI Key |
HMSYAPGFKGSXAJ-GFNGQHCCSA-N |
Isomeric SMILES |
CC[C@H](C(=O)NC/C=C/C=C(\C)/[C@H]([C@@H](C)[C@H]1[C@H]([C@H]([C@H](O1)/C=C/C=C/C=C(\C)/C(=O)C2=C(C=CNC2=O)O)O)O)OC)[C@@]3([C@@H]([C@@H](C([C@@H](O3)/C=C/C=C/C)(C)C)O)O)O |
SMILES |
CCC(C(=O)NCC=CC=C(C)C(C(C)C1C(C(C(O1)C=CC=CC=C(C)C(=O)C2=C(C=CNC2=O)O)O)O)OC)C3(C(C(C(C(O3)C=CC=CC)(C)C)O)O)O |
Canonical SMILES |
CCC(C(=O)NCC=CC=C(C)C(C(C)C1C(C(C(O1)C=CC=CC=C(C)C(=O)C2=C(C=CNC2=O)O)O)O)OC)C3(C(C(C(C(O3)C=CC=CC)(C)C)O)O)O |
Appearance |
Solid powder |
Purity |
>98% (or refer to the Certificate of Analysis) |
shelf_life |
>3 years if stored properly |
solubility |
Soluble in DMSO |
storage |
Dry, dark and at 0 - 4 C for short term (days to weeks) or -20 C for long term (months to years). |
Synonyms |
Kirromycin |
Origin of Product |
United States |
Retrosynthesis Analysis
AI-Powered Synthesis Planning: Our tool employs the Template_relevance Pistachio, Template_relevance Bkms_metabolic, Template_relevance Pistachio_ringbreaker, Template_relevance Reaxys, Template_relevance Reaxys_biocatalysis model, leveraging a vast database of chemical reactions to predict feasible synthetic routes.
One-Step Synthesis Focus: Specifically designed for one-step synthesis, it provides concise and direct routes for your target compounds, streamlining the synthesis process.
Accurate Predictions: Utilizing the extensive PISTACHIO, BKMS_METABOLIC, PISTACHIO_RINGBREAKER, REAXYS, REAXYS_BIOCATALYSIS database, our tool offers high-accuracy predictions, reflecting the latest in chemical research and data.
Strategy Settings
Precursor scoring | Relevance Heuristic |
---|---|
Min. plausibility | 0.01 |
Model | Template_relevance |
Template Set | Pistachio/Bkms_metabolic/Pistachio_ringbreaker/Reaxys/Reaxys_biocatalysis |
Top-N result to add to graph | 6 |
Feasible Synthetic Routes
Disclaimer and Information on In-Vitro Research Products
Please be aware that all articles and product information presented on BenchChem are intended solely for informational purposes. The products available for purchase on BenchChem are specifically designed for in-vitro studies, which are conducted outside of living organisms. In-vitro studies, derived from the Latin term "in glass," involve experiments performed in controlled laboratory settings using cells or tissues. It is important to note that these products are not categorized as medicines or drugs, and they have not received approval from the FDA for the prevention, treatment, or cure of any medical condition, ailment, or disease. We must emphasize that any form of bodily introduction of these products into humans or animals is strictly prohibited by law. It is essential to adhere to these guidelines to ensure compliance with legal and ethical standards in research and experimentation.