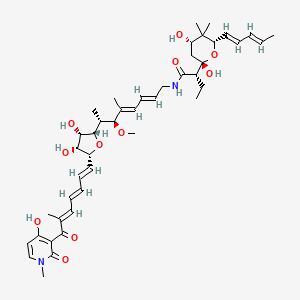
Heneicomycin
- Click on QUICK INQUIRY to receive a quote from our team of experts.
- With the quality product at a COMPETITIVE price, you can focus more on your research.
Overview
Description
Heneicomycin is an antibiotic compound that belongs to the elfamycin family. It was initially isolated from cultures of Streptomyces filipinensis and is known for its antibacterial properties. The molecular formula of this compound is C44H62N2O11 . It is structurally similar to other antibiotics in the elfamycin family, such as efrotomycin and mocimycin .
Preparation Methods
Synthetic Routes and Reaction Conditions
Heneicomycin is primarily produced through fermentation processes involving specific strains of Streptomyces. The strain Streptomyces sp. GE44282 is known to co-produce this compound and Aurodox . The bioconversion of this compound to Aurodox is substrate-specific and can be achieved using both growing and resting cells of Streptomyces sp. GE44282 .
Industrial Production Methods
Industrial production of this compound involves large-scale fermentation processes. The fermentation broth is subjected to various purification steps, including solvent extraction and chromatography, to isolate the antibiotic in its pure form .
Chemical Reactions Analysis
Types of Reactions
Heneicomycin undergoes several types of chemical reactions, including:
Reduction: Reduction reactions can modify the functional groups present in this compound, altering its antibacterial activity.
Substitution: Substitution reactions can replace specific functional groups in this compound, potentially leading to the formation of new derivatives with different properties.
Common Reagents and Conditions
Common reagents used in the chemical reactions of this compound include oxidizing agents like hydrogen peroxide and reducing agents like sodium borohydride. The reactions are typically carried out under controlled conditions, such as specific pH levels and temperatures, to ensure the desired outcomes .
Major Products Formed
The major product formed from the oxidation of this compound is Aurodox, which has enhanced antibacterial properties due to the presence of the hydroxyl group at the C30 position .
Scientific Research Applications
Mechanism of Action
Heneicomycin exerts its antibacterial effects by inhibiting the bacterial elongation factor Tu (EF-Tu), which is essential for protein synthesis in bacteria . By binding to EF-Tu, this compound prevents the proper functioning of the protein synthesis machinery, leading to the inhibition of bacterial growth and proliferation .
Comparison with Similar Compounds
Heneicomycin is structurally similar to other antibiotics in the elfamycin family, such as:
Mocimycin (Kirromycin): Mocimycin shares a similar mechanism of action with this compound but has different structural modifications that affect its activity.
X-5108 (Goldinomycin): Goldinomycin is another related compound with similar antibacterial properties but distinct structural features.
This compound’s uniqueness lies in its specific structural modifications, which contribute to its distinct antibacterial activity and potential for bioconversion to Aurodox .
Properties
IUPAC Name |
(2S)-2-[(2S,4S,6S)-2,4-dihydroxy-5,5-dimethyl-6-[(1E,3E)-penta-1,3-dienyl]oxan-2-yl]-N-[(2E,4E,6S,7R)-7-[(2S,3S,4R,5R)-3,4-dihydroxy-5-[(1E,3E,5E)-7-(4-hydroxy-1-methyl-2-oxopyridin-3-yl)-6-methyl-7-oxohepta-1,3,5-trienyl]oxolan-2-yl]-6-methoxy-5-methylocta-2,4-dienyl]butanamide |
Source
|
---|---|---|
Source | PubChem | |
URL | https://pubchem.ncbi.nlm.nih.gov | |
Description | Data deposited in or computed by PubChem | |
InChI |
InChI=1S/C44H62N2O11/c1-10-12-14-22-34-43(6,7)33(48)26-44(54,57-34)30(11-2)41(52)45-24-18-17-20-28(4)39(55-9)29(5)40-38(51)37(50)32(56-40)21-16-13-15-19-27(3)36(49)35-31(47)23-25-46(8)42(35)53/h10,12-23,25,29-30,32-34,37-40,47-48,50-51,54H,11,24,26H2,1-9H3,(H,45,52)/b12-10+,15-13+,18-17+,21-16+,22-14+,27-19+,28-20+/t29-,30-,32-,33+,34+,37+,38+,39-,40+,44+/m1/s1 |
Source
|
Source | PubChem | |
URL | https://pubchem.ncbi.nlm.nih.gov | |
Description | Data deposited in or computed by PubChem | |
InChI Key |
VKGDSUWMXCVJEA-ZODLGNRVSA-N |
Source
|
Source | PubChem | |
URL | https://pubchem.ncbi.nlm.nih.gov | |
Description | Data deposited in or computed by PubChem | |
Canonical SMILES |
CCC(C(=O)NCC=CC=C(C)C(C(C)C1C(C(C(O1)C=CC=CC=C(C)C(=O)C2=C(C=CN(C2=O)C)O)O)O)OC)C3(CC(C(C(O3)C=CC=CC)(C)C)O)O |
Source
|
Source | PubChem | |
URL | https://pubchem.ncbi.nlm.nih.gov | |
Description | Data deposited in or computed by PubChem | |
Isomeric SMILES |
CC[C@H](C(=O)NC/C=C/C=C(\C)/[C@H]([C@@H](C)[C@H]1[C@H]([C@H]([C@H](O1)/C=C/C=C/C=C(\C)/C(=O)C2=C(C=CN(C2=O)C)O)O)O)OC)[C@@]3(C[C@@H](C([C@@H](O3)/C=C/C=C/C)(C)C)O)O |
Source
|
Source | PubChem | |
URL | https://pubchem.ncbi.nlm.nih.gov | |
Description | Data deposited in or computed by PubChem | |
Molecular Formula |
C44H62N2O11 |
Source
|
Source | PubChem | |
URL | https://pubchem.ncbi.nlm.nih.gov | |
Description | Data deposited in or computed by PubChem | |
Molecular Weight |
795.0 g/mol |
Source
|
Source | PubChem | |
URL | https://pubchem.ncbi.nlm.nih.gov | |
Description | Data deposited in or computed by PubChem | |
CAS No. |
66170-37-4 |
Source
|
Record name | Heneicomycin | |
Source | ChemIDplus | |
URL | https://pubchem.ncbi.nlm.nih.gov/substance/?source=chemidplus&sourceid=0066170374 | |
Description | ChemIDplus is a free, web search system that provides access to the structure and nomenclature authority files used for the identification of chemical substances cited in National Library of Medicine (NLM) databases, including the TOXNET system. | |
Disclaimer and Information on In-Vitro Research Products
Please be aware that all articles and product information presented on BenchChem are intended solely for informational purposes. The products available for purchase on BenchChem are specifically designed for in-vitro studies, which are conducted outside of living organisms. In-vitro studies, derived from the Latin term "in glass," involve experiments performed in controlled laboratory settings using cells or tissues. It is important to note that these products are not categorized as medicines or drugs, and they have not received approval from the FDA for the prevention, treatment, or cure of any medical condition, ailment, or disease. We must emphasize that any form of bodily introduction of these products into humans or animals is strictly prohibited by law. It is essential to adhere to these guidelines to ensure compliance with legal and ethical standards in research and experimentation.