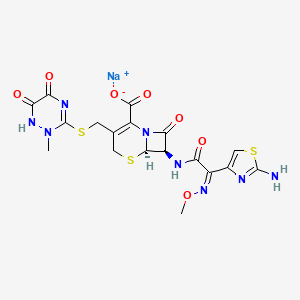
CEFTRIAXONE SODIUM
Overview
Description
Rocephin, known chemically as ceftriaxone, is a third-generation cephalosporin antibiotic. It is widely used to treat a variety of bacterial infections, including middle ear infections, endocarditis, meningitis, pneumonia, bone and joint infections, intra-abdominal infections, skin infections, urinary tract infections, gonorrhea, and pelvic inflammatory disease . Rocephin is administered either intravenously or intramuscularly and is known for its broad-spectrum activity against gram-negative bacteria .
Preparation Methods
Synthetic Routes and Reaction Conditions
Ceftriaxone is synthesized through a multi-step process that involves the formation of the beta-lactam ring, which is crucial for its antibiotic activity. The reaction conditions typically involve the use of organic solvents, controlled temperatures, and specific catalysts to ensure the correct formation of the desired chemical structure .
Industrial Production Methods
Industrial production of ceftriaxone involves large-scale fermentation processes to produce the 7-ACA core, followed by chemical modifications to introduce the necessary side chains. The final product is then purified through crystallization and filtration techniques to obtain a sterile, injectable form of the antibiotic .
Chemical Reactions Analysis
Types of Reactions
Ceftriaxone undergoes several types of chemical reactions, including:
Common Reagents and Conditions
Common reagents used in these reactions include oxidizing agents like hydrogen peroxide, reducing agents such as sodium borohydride, and various organic solvents. The reaction conditions often involve controlled temperatures, pH levels, and reaction times to achieve the desired outcomes .
Major Products Formed
The major products formed from these reactions include various ceftriaxone derivatives and degradation products, which can be analyzed to understand the stability and efficacy of the antibiotic under different conditions .
Scientific Research Applications
Ceftriaxone has a wide range of scientific research applications, including:
Chemistry: Used as a model compound to study beta-lactam antibiotics and their interactions with bacterial enzymes.
Biology: Investigated for its effects on bacterial cell wall synthesis and resistance mechanisms.
Industry: Employed in the development of new antibiotic formulations and delivery systems.
Mechanism of Action
Ceftriaxone exerts its effects by inhibiting bacterial cell wall synthesis. It binds to penicillin-binding proteins (PBPs) on the bacterial cell membrane, preventing the cross-linking of peptidoglycan chains, which is essential for cell wall integrity . This leads to the weakening of the cell wall and ultimately the lysis and death of the bacterial cell . The molecular targets of ceftriaxone include various PBPs, and its activity is enhanced by its ability to penetrate the outer membrane of gram-negative bacteria .
Comparison with Similar Compounds
Ceftriaxone is often compared with other cephalosporins and antibiotics, such as:
Cefotaxime: Another third-generation cephalosporin with similar antibacterial activity but a shorter half-life.
Cefepime: A fourth-generation cephalosporin with broader activity against gram-positive bacteria.
Ciprofloxacin: A fluoroquinolone antibiotic with a different mechanism of action, targeting bacterial DNA gyrase.
Ceftriaxone is unique in its long half-life, allowing for once-daily dosing, and its high penetration into the central nervous system, making it particularly effective for treating meningitis .
Properties
Key on ui mechanism of action |
Ceftriaxone works by inhibiting the mucopeptide synthesis in the bacterial cell wall. The beta-lactam moiety of ceftriaxone binds to carboxypeptidases, endopeptidases, and transpeptidases in the bacterial cytoplasmic membrane. These enzymes are involved in cell-wall synthesis and cell division. Binding of ceftriaxone to these enzymes causes the enzyme to lose activity; therefore, the bacteria produce defective cell walls, causing cell death. |
---|---|
CAS No. |
74578-69-1 |
Molecular Formula |
C18H17N8NaO7S3 |
Molecular Weight |
576.6 g/mol |
IUPAC Name |
sodium;7-[[2-(2-amino-1,3-thiazol-4-yl)-2-methoxyiminoacetyl]amino]-3-[(2-methyl-5,6-dioxo-1H-1,2,4-triazin-3-yl)sulfanylmethyl]-8-oxo-5-thia-1-azabicyclo[4.2.0]oct-2-ene-2-carboxylate |
InChI |
InChI=1S/C18H18N8O7S3.Na/c1-25-18(22-12(28)13(29)23-25)36-4-6-3-34-15-9(14(30)26(15)10(6)16(31)32)21-11(27)8(24-33-2)7-5-35-17(19)20-7;/h5,9,15H,3-4H2,1-2H3,(H2,19,20)(H,21,27)(H,23,29)(H,31,32);/q;+1/p-1 |
InChI Key |
YOBPSXOHCHDCMU-UHFFFAOYSA-M |
SMILES |
CN1C(=NC(=O)C(=O)N1)SCC2=C(N3C(C(C3=O)NC(=O)C(=NOC)C4=CSC(=N4)N)SC2)C(=O)[O-].[Na+] |
Isomeric SMILES |
CN1C(=NC(=O)C(=O)N1)SCC2=C(N3[C@@H]([C@@H](C3=O)NC(=O)/C(=N/OC)/C4=CSC(=N4)N)SC2)C(=O)O.[Na].[Na] |
Canonical SMILES |
CN1C(=NC(=O)C(=O)N1)SCC2=C(N3C(C(C3=O)NC(=O)C(=NOC)C4=CSC(=N4)N)SC2)C(=O)[O-].[Na+] |
Appearance |
White to light yellow solid powder. |
Color/Form |
Fluffy white powder White crystalline powde |
melting_point |
155 °C > 155 °C |
Key on ui other cas no. |
113852-37-2 149394-66-1 |
physical_description |
Solid |
Pictograms |
Irritant; Health Hazard |
Purity |
>98% (or refer to the Certificate of Analysis) |
Related CAS |
104376-79-6 (di-hydrochloride salt) 74578-69-1 (di-hydrochloride salt, hemiheptahydrate) |
shelf_life |
It is recommended that cidofovir admixtures be administered within 24 hours of preparation and that refrigeration or freezer storage not be used to extend this 24 hour limit. |
solubility |
1.05e-01 g/L |
storage |
Dry, dark and at 0 - 4 C for short term (days to weeks) or -20 C for long term (months to years). |
Synonyms |
Anhydrous Ceftriaxone Sodium Benaxona Cefatriaxone Cefaxona Ceftrex Ceftriaxon Ceftriaxon Curamed Ceftriaxon Hexal Ceftriaxona Andreu Ceftriaxona LDP Torlan Ceftriaxone Ceftriaxone Irex Ceftriaxone Sodium Ceftriaxone Sodium, Anhydrous Ceftriaxone, Disodium Salt Ceftriaxone, Disodium Salt, Hemiheptahydrate Lendacin Longacef Longaceph Ro 13 9904 Ro 13-9904 Ro 139904 Ro-13-9904 Ro13 9904 Ro13-9904 Ro139904 Rocefalin Rocefin Rocephin Rocephine Tacex Terbac |
Origin of Product |
United States |
Retrosynthesis Analysis
AI-Powered Synthesis Planning: Our tool employs the Template_relevance Pistachio, Template_relevance Bkms_metabolic, Template_relevance Pistachio_ringbreaker, Template_relevance Reaxys, Template_relevance Reaxys_biocatalysis model, leveraging a vast database of chemical reactions to predict feasible synthetic routes.
One-Step Synthesis Focus: Specifically designed for one-step synthesis, it provides concise and direct routes for your target compounds, streamlining the synthesis process.
Accurate Predictions: Utilizing the extensive PISTACHIO, BKMS_METABOLIC, PISTACHIO_RINGBREAKER, REAXYS, REAXYS_BIOCATALYSIS database, our tool offers high-accuracy predictions, reflecting the latest in chemical research and data.
Strategy Settings
Precursor scoring | Relevance Heuristic |
---|---|
Min. plausibility | 0.01 |
Model | Template_relevance |
Template Set | Pistachio/Bkms_metabolic/Pistachio_ringbreaker/Reaxys/Reaxys_biocatalysis |
Top-N result to add to graph | 6 |
Feasible Synthetic Routes
Disclaimer and Information on In-Vitro Research Products
Please be aware that all articles and product information presented on BenchChem are intended solely for informational purposes. The products available for purchase on BenchChem are specifically designed for in-vitro studies, which are conducted outside of living organisms. In-vitro studies, derived from the Latin term "in glass," involve experiments performed in controlled laboratory settings using cells or tissues. It is important to note that these products are not categorized as medicines or drugs, and they have not received approval from the FDA for the prevention, treatment, or cure of any medical condition, ailment, or disease. We must emphasize that any form of bodily introduction of these products into humans or animals is strictly prohibited by law. It is essential to adhere to these guidelines to ensure compliance with legal and ethical standards in research and experimentation.