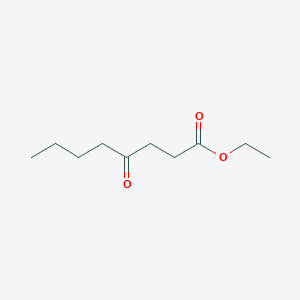
Ethyl 4-oxooctanoate
Overview
Description
Ethyl 4-oxooctanoate is an organic compound with the molecular formula C10H18O3 and a molecular weight of 186.25 g/mol . It is a liquid at room temperature and is known for its applications in various chemical reactions and industrial processes.
Preparation Methods
Synthetic Routes and Reaction Conditions
Ethyl 4-oxooctanoate can be synthesized through the reaction of ethyl 4-chloro-4-oxobutanoate with n-butylmagnesium chloride in the presence of a catalytic amount of tris(acetylacetonato)iron(III) in tetrahydrofuran at -29°C . The reaction is quenched with dilute hydrochloric acid, followed by work-up and purification to yield this compound.
Industrial Production Methods
Industrial production methods for this compound typically involve similar synthetic routes but on a larger scale, ensuring high yield and purity. The use of efficient catalysts and optimized reaction conditions are crucial for large-scale production.
Chemical Reactions Analysis
Types of Reactions
Ethyl 4-oxooctanoate undergoes various chemical reactions, including condensation reactions with amines . These reactions can lead to the formation of amides and other derivatives.
Common Reagents and Conditions
Common reagents used in reactions with this compound include amines, Grignard reagents, and catalytic amounts of transition metal complexes. Reaction conditions often involve controlled temperatures and specific solvents to ensure high yields and selectivity.
Major Products Formed
The major products formed from reactions involving this compound include amides, esters, and other functionalized derivatives. These products are valuable intermediates in the synthesis of more complex molecules.
Scientific Research Applications
Ethyl 4-oxooctanoate is used in various scientific research applications, including:
Chemistry: As a reagent in organic synthesis and as an intermediate in the preparation of more complex molecules.
Biology: In the study of enzyme-catalyzed reactions and metabolic pathways.
Medicine: As a precursor in the synthesis of pharmaceutical compounds.
Industry: In the production of specialty chemicals and materials.
Mechanism of Action
The mechanism by which ethyl 4-oxooctanoate exerts its effects involves its reactivity with nucleophiles, such as amines, to form amides and other derivatives. The molecular targets and pathways involved in these reactions are primarily related to the functional groups present in the compound, such as the ester and ketone groups .
Comparison with Similar Compounds
Similar Compounds
Similar compounds to ethyl 4-oxooctanoate include:
- Ethyl 4-chloro-4-oxobutanoate
- Ethyl 4-aminobutyrate
- Ethyl 4-hydroxybutanoate
Uniqueness
This compound is unique due to its specific structure, which allows it to undergo a variety of chemical reactions, making it a versatile intermediate in organic synthesis. Its reactivity with different nucleophiles and its ability to form stable products highlight its importance in both research and industrial applications .
Properties
IUPAC Name |
ethyl 4-oxooctanoate | |
---|---|---|
Source | PubChem | |
URL | https://pubchem.ncbi.nlm.nih.gov | |
Description | Data deposited in or computed by PubChem | |
InChI |
InChI=1S/C10H18O3/c1-3-5-6-9(11)7-8-10(12)13-4-2/h3-8H2,1-2H3 | |
Source | PubChem | |
URL | https://pubchem.ncbi.nlm.nih.gov | |
Description | Data deposited in or computed by PubChem | |
InChI Key |
IKNPZDWWOFUFHY-UHFFFAOYSA-N | |
Source | PubChem | |
URL | https://pubchem.ncbi.nlm.nih.gov | |
Description | Data deposited in or computed by PubChem | |
Canonical SMILES |
CCCCC(=O)CCC(=O)OCC | |
Source | PubChem | |
URL | https://pubchem.ncbi.nlm.nih.gov | |
Description | Data deposited in or computed by PubChem | |
Molecular Formula |
C10H18O3 | |
Source | PubChem | |
URL | https://pubchem.ncbi.nlm.nih.gov | |
Description | Data deposited in or computed by PubChem | |
DSSTOX Substance ID |
DTXSID10474051 | |
Record name | ethyl 4-oxooctanoate | |
Source | EPA DSSTox | |
URL | https://comptox.epa.gov/dashboard/DTXSID10474051 | |
Description | DSSTox provides a high quality public chemistry resource for supporting improved predictive toxicology. | |
Molecular Weight |
186.25 g/mol | |
Source | PubChem | |
URL | https://pubchem.ncbi.nlm.nih.gov | |
Description | Data deposited in or computed by PubChem | |
CAS No. |
37174-96-2 | |
Record name | ethyl 4-oxooctanoate | |
Source | EPA DSSTox | |
URL | https://comptox.epa.gov/dashboard/DTXSID10474051 | |
Description | DSSTox provides a high quality public chemistry resource for supporting improved predictive toxicology. | |
Retrosynthesis Analysis
AI-Powered Synthesis Planning: Our tool employs the Template_relevance Pistachio, Template_relevance Bkms_metabolic, Template_relevance Pistachio_ringbreaker, Template_relevance Reaxys, Template_relevance Reaxys_biocatalysis model, leveraging a vast database of chemical reactions to predict feasible synthetic routes.
One-Step Synthesis Focus: Specifically designed for one-step synthesis, it provides concise and direct routes for your target compounds, streamlining the synthesis process.
Accurate Predictions: Utilizing the extensive PISTACHIO, BKMS_METABOLIC, PISTACHIO_RINGBREAKER, REAXYS, REAXYS_BIOCATALYSIS database, our tool offers high-accuracy predictions, reflecting the latest in chemical research and data.
Strategy Settings
Precursor scoring | Relevance Heuristic |
---|---|
Min. plausibility | 0.01 |
Model | Template_relevance |
Template Set | Pistachio/Bkms_metabolic/Pistachio_ringbreaker/Reaxys/Reaxys_biocatalysis |
Top-N result to add to graph | 6 |
Feasible Synthetic Routes
Disclaimer and Information on In-Vitro Research Products
Please be aware that all articles and product information presented on BenchChem are intended solely for informational purposes. The products available for purchase on BenchChem are specifically designed for in-vitro studies, which are conducted outside of living organisms. In-vitro studies, derived from the Latin term "in glass," involve experiments performed in controlled laboratory settings using cells or tissues. It is important to note that these products are not categorized as medicines or drugs, and they have not received approval from the FDA for the prevention, treatment, or cure of any medical condition, ailment, or disease. We must emphasize that any form of bodily introduction of these products into humans or animals is strictly prohibited by law. It is essential to adhere to these guidelines to ensure compliance with legal and ethical standards in research and experimentation.