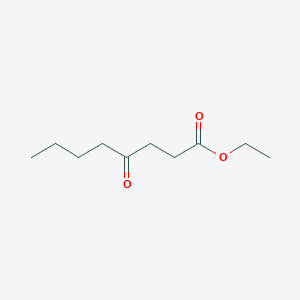
Ethyl 4-oxooctanoate
Vue d'ensemble
Description
Ethyl 4-oxooctanoate, also known as 4-ethyloctanoic acid ethyl ester, is a naturally occurring fatty acid ester found in various fruits, vegetables, and oils. It is a colorless, odorless, and slightly viscous liquid at room temperature. Its chemical formula is C10H20O3. This compound has a wide range of applications in the food, cosmetics, and pharmaceutical industries due to its unique properties.
Applications De Recherche Scientifique
Chemoenzymatic Synthesis
Ethyl 2-hydroxy-3-oxooctanoate, closely related to Ethyl 4-oxooctanoate, has been utilized in the chemoenzymatic synthesis of various compounds. A study by Fadnavis, Vadivel, and Sharfuddin (1999) describes the reduction of this compound using immobilized baker's yeast, leading to the production of high diastereoselectivity and enantioselectivity compounds such as (4S,5R)-5-hydroxy-γ-decalactone (Fadnavis et al., 1999).
Asymmetric Synthesis
This compound derivatives have been used in asymmetric synthesis. For instance, Tsuboi, Furutani, and Takeda (1987) demonstrated the asymmetric reduction of ethyl 3-chloro-2-oxooctanoate, a similar compound, to produce key intermediates for complex molecules such as leukotriene A4 (Tsuboi et al., 1987).
Synthetic Studies and Structural Assignments
In the field of organic chemistry, this compound and its derivatives play a critical role in the synthesis and structural determination of complex molecules. Ashton and Doss (1993) used ethyl 2,4-dioxooctanoate for the synthesis of various carboxylates, demonstrating its versatility in organic synthesis (Ashton & Doss, 1993).
Enzyme-Catalyzed Asymmetric Reduction
This compound derivatives have been subject to enzyme-catalyzed asymmetric reduction. Shimizu et al. (1990) explored the reduction of ethyl 4-chloro-3-oxobutanoate using microbial aldehyde reductase, showing its potential in producing enantiomerically pure compounds (Shimizu et al., 1990).
Large Scale Preparation and Utilization
This compound is also significant in large-scale chemical synthesis. Magano, Nanninga, and Winkle (2008) developed an efficient method for converting N-acyl oxazolidinones to β-keto ester, highlighting the compound's utility in large-scale preparations (Magano et al., 2008).
Safety and Hazards
Ethyl 4-oxooctanoate is classified as a flammable liquid (Category 4), and it poses a short-term (acute) aquatic hazard (Category 2) and a long-term (chronic) aquatic hazard (Category 2). It is recommended to avoid dust formation, breathing mist, gas or vapours, and contact with skin and eyes. Use personal protective equipment, wear chemical impermeable gloves, and ensure adequate ventilation .
Mécanisme D'action
Target of Action
Ethyl 4-oxooctanoate is a chemical compound with the molecular formula C10H18O3
Biochemical Pathways
It has been used in condensation reactions with amines . The downstream effects of these reactions and their implications on biological systems warrant further investigation.
Result of Action
It has been used in condensation reactions with amines, and the product was recovered in 88% yield after purification
Analyse Biochimique
Biochemical Properties
Ethyl 4-oxooctanoate plays a significant role in biochemical reactions, particularly in the context of fatty acid metabolism. It interacts with enzymes such as acyl-CoA dehydrogenase and enoyl-CoA hydratase, which are involved in the β-oxidation pathway. These interactions facilitate the breakdown of fatty acids into acetyl-CoA units, which are then utilized in the citric acid cycle for energy production. The nature of these interactions involves the binding of this compound to the active sites of these enzymes, leading to the formation of enzyme-substrate complexes that undergo subsequent catalytic transformations .
Cellular Effects
This compound influences various cellular processes, including cell signaling pathways, gene expression, and cellular metabolism. In particular, it has been observed to modulate the activity of peroxisome proliferator-activated receptors (PPARs), which are nuclear receptors that regulate the expression of genes involved in lipid metabolism. By activating PPARs, this compound can enhance the expression of genes encoding enzymes responsible for fatty acid oxidation, thereby promoting lipid catabolism and energy production . Additionally, this compound can affect cell signaling pathways related to inflammation and oxidative stress, potentially offering protective effects against cellular damage.
Molecular Mechanism
The molecular mechanism of action of this compound involves its binding interactions with specific biomolecules. For instance, it can act as a ligand for PPARs, leading to the activation of these receptors and subsequent transcriptional regulation of target genes. Furthermore, this compound can inhibit the activity of certain enzymes, such as acetyl-CoA carboxylase, which plays a crucial role in fatty acid synthesis. By inhibiting this enzyme, this compound reduces the synthesis of fatty acids, thereby shifting the cellular metabolic balance towards increased fatty acid oxidation .
Temporal Effects in Laboratory Settings
In laboratory settings, the effects of this compound can change over time due to its stability and degradation properties. Studies have shown that this compound is relatively stable under standard laboratory conditions, with minimal degradation observed over extended periods. Its long-term effects on cellular function can vary depending on the experimental conditions. For example, prolonged exposure to this compound in cell culture models has been associated with sustained activation of PPARs and enhanced fatty acid oxidation . In in vivo studies, the compound’s stability and bioavailability can influence its temporal effects on metabolic processes.
Dosage Effects in Animal Models
The effects of this compound in animal models vary with different dosages. At low to moderate doses, the compound has been shown to enhance lipid metabolism and improve energy homeostasis without causing significant adverse effects. At high doses, this compound can exhibit toxic effects, including hepatotoxicity and oxidative stress . These threshold effects highlight the importance of dose optimization in experimental studies to achieve the desired biochemical outcomes while minimizing potential toxicity.
Metabolic Pathways
This compound is involved in several metabolic pathways, primarily related to fatty acid metabolism. It serves as a substrate for enzymes in the β-oxidation pathway, leading to the production of acetyl-CoA units that enter the citric acid cycle. Additionally, this compound can influence the activity of enzymes involved in fatty acid synthesis and degradation, thereby modulating metabolic flux and metabolite levels . The compound’s interactions with cofactors such as NAD+ and FAD further facilitate its role in these metabolic pathways.
Transport and Distribution
Within cells and tissues, this compound is transported and distributed through interactions with specific transporters and binding proteins. For instance, it can be taken up by cells via fatty acid transport proteins (FATPs) and subsequently bind to intracellular lipid-binding proteins (LBPs) that facilitate its distribution to various cellular compartments . These interactions ensure the efficient transport and localization of this compound, enabling its participation in metabolic processes.
Subcellular Localization
The subcellular localization of this compound is primarily within the mitochondria, where it participates in fatty acid oxidation. The compound’s targeting to mitochondria is facilitated by specific signals and post-translational modifications that direct its transport to this organelle . Within the mitochondria, this compound undergoes enzymatic transformations that contribute to energy production and metabolic regulation.
Propriétés
IUPAC Name |
ethyl 4-oxooctanoate | |
---|---|---|
Source | PubChem | |
URL | https://pubchem.ncbi.nlm.nih.gov | |
Description | Data deposited in or computed by PubChem | |
InChI |
InChI=1S/C10H18O3/c1-3-5-6-9(11)7-8-10(12)13-4-2/h3-8H2,1-2H3 | |
Source | PubChem | |
URL | https://pubchem.ncbi.nlm.nih.gov | |
Description | Data deposited in or computed by PubChem | |
InChI Key |
IKNPZDWWOFUFHY-UHFFFAOYSA-N | |
Source | PubChem | |
URL | https://pubchem.ncbi.nlm.nih.gov | |
Description | Data deposited in or computed by PubChem | |
Canonical SMILES |
CCCCC(=O)CCC(=O)OCC | |
Source | PubChem | |
URL | https://pubchem.ncbi.nlm.nih.gov | |
Description | Data deposited in or computed by PubChem | |
Molecular Formula |
C10H18O3 | |
Source | PubChem | |
URL | https://pubchem.ncbi.nlm.nih.gov | |
Description | Data deposited in or computed by PubChem | |
DSSTOX Substance ID |
DTXSID10474051 | |
Record name | ethyl 4-oxooctanoate | |
Source | EPA DSSTox | |
URL | https://comptox.epa.gov/dashboard/DTXSID10474051 | |
Description | DSSTox provides a high quality public chemistry resource for supporting improved predictive toxicology. | |
Molecular Weight |
186.25 g/mol | |
Source | PubChem | |
URL | https://pubchem.ncbi.nlm.nih.gov | |
Description | Data deposited in or computed by PubChem | |
CAS RN |
37174-96-2 | |
Record name | ethyl 4-oxooctanoate | |
Source | EPA DSSTox | |
URL | https://comptox.epa.gov/dashboard/DTXSID10474051 | |
Description | DSSTox provides a high quality public chemistry resource for supporting improved predictive toxicology. | |
Retrosynthesis Analysis
AI-Powered Synthesis Planning: Our tool employs the Template_relevance Pistachio, Template_relevance Bkms_metabolic, Template_relevance Pistachio_ringbreaker, Template_relevance Reaxys, Template_relevance Reaxys_biocatalysis model, leveraging a vast database of chemical reactions to predict feasible synthetic routes.
One-Step Synthesis Focus: Specifically designed for one-step synthesis, it provides concise and direct routes for your target compounds, streamlining the synthesis process.
Accurate Predictions: Utilizing the extensive PISTACHIO, BKMS_METABOLIC, PISTACHIO_RINGBREAKER, REAXYS, REAXYS_BIOCATALYSIS database, our tool offers high-accuracy predictions, reflecting the latest in chemical research and data.
Strategy Settings
Precursor scoring | Relevance Heuristic |
---|---|
Min. plausibility | 0.01 |
Model | Template_relevance |
Template Set | Pistachio/Bkms_metabolic/Pistachio_ringbreaker/Reaxys/Reaxys_biocatalysis |
Top-N result to add to graph | 6 |
Feasible Synthetic Routes
Avertissement et informations sur les produits de recherche in vitro
Veuillez noter que tous les articles et informations sur les produits présentés sur BenchChem sont destinés uniquement à des fins informatives. Les produits disponibles à l'achat sur BenchChem sont spécifiquement conçus pour des études in vitro, qui sont réalisées en dehors des organismes vivants. Les études in vitro, dérivées du terme latin "in verre", impliquent des expériences réalisées dans des environnements de laboratoire contrôlés à l'aide de cellules ou de tissus. Il est important de noter que ces produits ne sont pas classés comme médicaments et n'ont pas reçu l'approbation de la FDA pour la prévention, le traitement ou la guérison de toute condition médicale, affection ou maladie. Nous devons souligner que toute forme d'introduction corporelle de ces produits chez les humains ou les animaux est strictement interdite par la loi. Il est essentiel de respecter ces directives pour assurer la conformité aux normes légales et éthiques en matière de recherche et d'expérimentation.