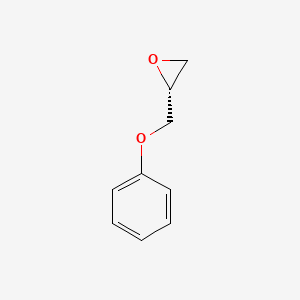
(R)-Glycidyl phenyl ether
Overview
Description
®-Glycidyl phenyl ether is an organic compound that belongs to the class of epoxides. It is characterized by the presence of an epoxide ring and a phenyl group attached to the glycidyl moiety. This compound is known for its reactivity and versatility in various chemical reactions, making it valuable in different fields of scientific research and industrial applications.
Mechanism of Action
Target of Action
The primary target of ®-Glycidyl phenyl ether is enzymes involved in primary metabolic pathways . These enzymes play crucial roles in various biochemical reactions within the cell. The interaction of ®-Glycidyl phenyl ether with these enzymes can lead to significant changes in cellular processes .
Mode of Action
®-Glycidyl phenyl ether interacts with its targets primarily through a process known as acidic cleavage . During this process, the ether oxygen in the compound is protonated, forming a good leaving group. This group can then be eliminated as part of an S_N2, S_N1, or E1 reaction mechanism . The exact pathway is determined by the strong acid used and the type of substituents attached to the ether .
Biochemical Pathways
The interaction of ®-Glycidyl phenyl ether with its targets affects several biochemical pathways. One of the key pathways influenced is the phenylpropane pathway . This pathway is strongly enhanced under certain conditions, such as salt stress . The phenylpropane pathway is involved in the synthesis of essential oils, which are major secondary metabolites of aromatic plants .
Pharmacokinetics
Ethers in general have a small dipole moment due to the presence of an electronegative oxygen atom . This could potentially influence the compound’s bioavailability.
Result of Action
The molecular and cellular effects of ®-Glycidyl phenyl ether’s action are largely dependent on its interaction with its targets and the subsequent changes in biochemical pathways. For instance, the enhancement of the phenylpropane pathway could lead to an increase in the production of essential oils . Additionally, the compound’s interaction with enzymes could lead to significant changes in cellular processes .
Action Environment
Environmental factors can significantly influence the action, efficacy, and stability of ®-Glycidyl phenyl ether. Factors such as light, temperature, drought, salinity, heavy metals, seasonal variations, and mineral concentrations can have a significant impact on the chemical nature and stability of essential oil components . These factors can also regulate the biosynthesis and yield of essential oils .
Biochemical Analysis
Biochemical Properties
®-Glycidyl phenyl ether plays a significant role in biochemical reactions due to its ability to interact with various enzymes and proteins. One of the key interactions is with epoxide hydrolases, which catalyze the enantioselective ring opening of ®-glycidyl phenyl ether to produce enantiopure vicinal diols . This interaction is crucial for the synthesis of chiral drug intermediates. Additionally, ®-glycidyl phenyl ether can undergo anionic polymerization, further highlighting its versatility in biochemical processes .
Cellular Effects
®-Glycidyl phenyl ether has notable effects on various types of cells and cellular processes. It has been observed to influence cell signaling pathways, gene expression, and cellular metabolism. For instance, in Bacillus megaterium, ®-glycidyl phenyl ether showed high enantioselectivity and enhanced epoxide hydrolase activity when used with specific cosolvents . This indicates its potential impact on cellular functions and metabolic processes.
Molecular Mechanism
The molecular mechanism of ®-Glycidyl phenyl ether involves its interaction with biomolecules, leading to enzyme inhibition or activation and changes in gene expression. The compound’s epoxide group is highly reactive, allowing it to form covalent bonds with nucleophilic sites on enzymes and proteins. This can result in the inhibition of enzyme activity or the activation of specific biochemical pathways . Additionally, ®-glycidyl phenyl ether can induce changes in gene expression by interacting with transcription factors and other regulatory proteins.
Temporal Effects in Laboratory Settings
In laboratory settings, the effects of ®-Glycidyl phenyl ether can change over time due to its stability and degradation. The compound is known to be air-sensitive and should be stored under inert gas to maintain its stability . Over time, ®-glycidyl phenyl ether may degrade, leading to changes in its biochemical activity and long-term effects on cellular function. Studies have shown that prolonged exposure to ®-glycidyl phenyl ether can cause damage to organs through repeated exposure .
Dosage Effects in Animal Models
The effects of ®-Glycidyl phenyl ether vary with different dosages in animal models. At lower doses, the compound may exhibit beneficial effects, such as enhanced enzyme activity and improved metabolic processes. At higher doses, ®-glycidyl phenyl ether can cause toxic or adverse effects, including skin irritation, respiratory irritation, and potential damage to reproductive organs . It is important to carefully control the dosage to avoid these adverse effects.
Metabolic Pathways
®-Glycidyl phenyl ether is involved in various metabolic pathways, interacting with enzymes and cofactors that facilitate its conversion into other metabolites. The compound can undergo enzymatic hydrolysis by epoxide hydrolases, resulting in the formation of diols . These diols can then enter further metabolic pathways, contributing to the overall metabolic flux and affecting metabolite levels in cells.
Transport and Distribution
Within cells and tissues, ®-Glycidyl phenyl ether is transported and distributed through interactions with transporters and binding proteins. The compound’s lipophilic nature allows it to easily cross cell membranes and accumulate in specific cellular compartments . This distribution can influence its localization and accumulation, affecting its biochemical activity and interactions with other biomolecules.
Subcellular Localization
The subcellular localization of ®-Glycidyl phenyl ether is influenced by targeting signals and post-translational modifications that direct it to specific compartments or organelles. The compound has been observed to localize in the endoplasmic reticulum and other membrane-bound organelles, where it can interact with enzymes and proteins involved in various biochemical processes . This localization is crucial for its activity and function within cells.
Preparation Methods
Synthetic Routes and Reaction Conditions
®-Glycidyl phenyl ether can be synthesized through several methods. One common approach is the epoxidation of allyl phenyl ether using a peracid, such as m-chloroperoxybenzoic acid, under controlled conditions. The reaction typically involves the addition of the peracid to the allyl phenyl ether in an inert solvent, followed by stirring at a specific temperature to achieve the desired product.
Another method involves the reaction of phenol with epichlorohydrin in the presence of a base, such as sodium hydroxide. This reaction proceeds through the formation of an intermediate, which undergoes intramolecular cyclization to form the epoxide ring.
Industrial Production Methods
In industrial settings, ®-Glycidyl phenyl ether is often produced using large-scale epoxidation processes. These processes involve the use of continuous reactors and optimized reaction conditions to ensure high yield and purity of the product. The choice of catalysts, solvents, and reaction parameters is crucial in achieving efficient and cost-effective production.
Chemical Reactions Analysis
Types of Reactions
®-Glycidyl phenyl ether undergoes various types of chemical reactions, including:
Oxidation: The epoxide ring can be opened by oxidizing agents, leading to the formation of diols or other oxygenated products.
Reduction: Reduction reactions can convert the epoxide ring into an alcohol or other reduced forms.
Substitution: The epoxide ring can be substituted by nucleophiles, resulting in the formation of different functionalized products.
Common Reagents and Conditions
Oxidation: Common oxidizing agents include hydrogen peroxide, peracids, and osmium tetroxide. These reactions are typically carried out in the presence of a solvent, such as dichloromethane or acetonitrile, under controlled temperature and pressure conditions.
Reduction: Reducing agents like lithium aluminum hydride or sodium borohydride are used to reduce the epoxide ring. These reactions are often conducted in anhydrous solvents, such as tetrahydrofuran or diethyl ether.
Substitution: Nucleophiles, such as amines, thiols, or halides, can react with the epoxide ring under basic or acidic conditions to form substituted products.
Major Products Formed
The major products formed from these reactions depend on the specific reagents and conditions used. For example, oxidation of ®-Glycidyl phenyl ether can yield diols, while reduction can produce alcohols. Substitution reactions can lead to a variety of functionalized compounds, depending on the nucleophile employed.
Scientific Research Applications
®-Glycidyl phenyl ether has a wide range of scientific research applications, including:
Chemistry: It is used as a building block in the synthesis of complex organic molecules and polymers. Its reactivity makes it valuable in various organic transformations and catalysis.
Biology: The compound is employed in the modification of biomolecules, such as proteins and nucleic acids, for studying their structure and function.
Medicine: ®-Glycidyl phenyl ether is used in the development of pharmaceutical intermediates and active pharmaceutical ingredients. Its ability to form covalent bonds with biological targets makes it useful in drug design and discovery.
Industry: The compound is utilized in the production of epoxy resins, coatings, adhesives, and other materials. Its reactivity and stability contribute to the performance and durability of these products.
Comparison with Similar Compounds
Similar Compounds
- Glycidyl methyl ether
- Glycidyl ethyl ether
- Glycidyl butyl ether
- Phenyl glycidyl ether
Uniqueness
®-Glycidyl phenyl ether is unique due to the presence of both the epoxide ring and the phenyl group. This combination imparts distinct reactivity and properties compared to other glycidyl ethers. The phenyl group enhances the compound’s stability and provides additional sites for functionalization, making it more versatile in various applications.
Properties
IUPAC Name |
(2R)-2-(phenoxymethyl)oxirane | |
---|---|---|
Source | PubChem | |
URL | https://pubchem.ncbi.nlm.nih.gov | |
Description | Data deposited in or computed by PubChem | |
InChI |
InChI=1S/C9H10O2/c1-2-4-8(5-3-1)10-6-9-7-11-9/h1-5,9H,6-7H2/t9-/m0/s1 | |
Source | PubChem | |
URL | https://pubchem.ncbi.nlm.nih.gov | |
Description | Data deposited in or computed by PubChem | |
InChI Key |
FQYUMYWMJTYZTK-VIFPVBQESA-N | |
Source | PubChem | |
URL | https://pubchem.ncbi.nlm.nih.gov | |
Description | Data deposited in or computed by PubChem | |
Canonical SMILES |
C1C(O1)COC2=CC=CC=C2 | |
Source | PubChem | |
URL | https://pubchem.ncbi.nlm.nih.gov | |
Description | Data deposited in or computed by PubChem | |
Isomeric SMILES |
C1[C@@H](O1)COC2=CC=CC=C2 | |
Source | PubChem | |
URL | https://pubchem.ncbi.nlm.nih.gov | |
Description | Data deposited in or computed by PubChem | |
Molecular Formula |
C9H10O2 | |
Source | PubChem | |
URL | https://pubchem.ncbi.nlm.nih.gov | |
Description | Data deposited in or computed by PubChem | |
DSSTOX Substance ID |
DTXSID901308155 | |
Record name | (2R)-2-(Phenoxymethyl)oxirane | |
Source | EPA DSSTox | |
URL | https://comptox.epa.gov/dashboard/DTXSID901308155 | |
Description | DSSTox provides a high quality public chemistry resource for supporting improved predictive toxicology. | |
Molecular Weight |
150.17 g/mol | |
Source | PubChem | |
URL | https://pubchem.ncbi.nlm.nih.gov | |
Description | Data deposited in or computed by PubChem | |
CAS No. |
71031-02-2 | |
Record name | (2R)-2-(Phenoxymethyl)oxirane | |
Source | CAS Common Chemistry | |
URL | https://commonchemistry.cas.org/detail?cas_rn=71031-02-2 | |
Description | CAS Common Chemistry is an open community resource for accessing chemical information. Nearly 500,000 chemical substances from CAS REGISTRY cover areas of community interest, including common and frequently regulated chemicals, and those relevant to high school and undergraduate chemistry classes. This chemical information, curated by our expert scientists, is provided in alignment with our mission as a division of the American Chemical Society. | |
Explanation | The data from CAS Common Chemistry is provided under a CC-BY-NC 4.0 license, unless otherwise stated. | |
Record name | (2R)-2-(Phenoxymethyl)oxirane | |
Source | EPA DSSTox | |
URL | https://comptox.epa.gov/dashboard/DTXSID901308155 | |
Description | DSSTox provides a high quality public chemistry resource for supporting improved predictive toxicology. | |
Synthesis routes and methods I
Procedure details
Synthesis routes and methods II
Procedure details
Synthesis routes and methods III
Procedure details
Synthesis routes and methods IV
Procedure details
Retrosynthesis Analysis
AI-Powered Synthesis Planning: Our tool employs the Template_relevance Pistachio, Template_relevance Bkms_metabolic, Template_relevance Pistachio_ringbreaker, Template_relevance Reaxys, Template_relevance Reaxys_biocatalysis model, leveraging a vast database of chemical reactions to predict feasible synthetic routes.
One-Step Synthesis Focus: Specifically designed for one-step synthesis, it provides concise and direct routes for your target compounds, streamlining the synthesis process.
Accurate Predictions: Utilizing the extensive PISTACHIO, BKMS_METABOLIC, PISTACHIO_RINGBREAKER, REAXYS, REAXYS_BIOCATALYSIS database, our tool offers high-accuracy predictions, reflecting the latest in chemical research and data.
Strategy Settings
Precursor scoring | Relevance Heuristic |
---|---|
Min. plausibility | 0.01 |
Model | Template_relevance |
Template Set | Pistachio/Bkms_metabolic/Pistachio_ringbreaker/Reaxys/Reaxys_biocatalysis |
Top-N result to add to graph | 6 |
Feasible Synthetic Routes
Q1: How does the addition of Tween-80 affect the enzymatic activity of Bacillus megaterium epoxide hydrolase towards (R)-Glycidyl phenyl ether?
A1: The research paper demonstrates that the presence of Tween-80, a nonionic surfactant, significantly enhances the activity of Bacillus megaterium epoxide hydrolase towards this compound []. This enhancement manifests as a nearly two-fold increase in the enzyme's apparent activity compared to when DMSO is used as a co-solvent. The researchers suggest that this improvement likely stems from Tween-80's ability to create an emulsion, improving substrate availability to the enzyme. Additionally, Tween-80 positively influences the enzyme's thermostability, increasing its half-life at 50°C by 2.5 times [].
Disclaimer and Information on In-Vitro Research Products
Please be aware that all articles and product information presented on BenchChem are intended solely for informational purposes. The products available for purchase on BenchChem are specifically designed for in-vitro studies, which are conducted outside of living organisms. In-vitro studies, derived from the Latin term "in glass," involve experiments performed in controlled laboratory settings using cells or tissues. It is important to note that these products are not categorized as medicines or drugs, and they have not received approval from the FDA for the prevention, treatment, or cure of any medical condition, ailment, or disease. We must emphasize that any form of bodily introduction of these products into humans or animals is strictly prohibited by law. It is essential to adhere to these guidelines to ensure compliance with legal and ethical standards in research and experimentation.