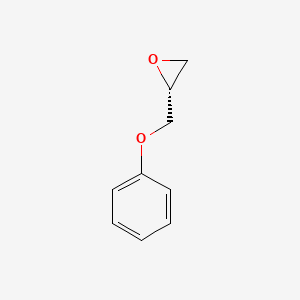
(R)-苯甲基缩水甘油醚
概述
描述
(R)-Glycidyl phenyl ether, also known as (R)-GPE, is an important organic compound used in many scientific research applications. It is a chiral ether with a phenyl group attached to the oxygen atom, and is used as a synthetic intermediate in organic chemistry. This compound has been studied extensively in the laboratory, and its structure and properties have been well-characterized. In recent years, (R)-GPE has been used in various scientific research applications, including chemical synthesis, drug development, and biotechnology.
科学研究应用
酶催化的动力学拆分:Bendigiri 等人 (2018 年) 研究了来自假丝酵母的环氧化物水解酶 Ylehd,以了解其在苯基缩水甘油醚衍生物动力学拆分中的对映选择性。他们发现,该酶可以选择性地将苯甲基缩水甘油醚的特定对映异构体转化为其相应的二醇,保持高对映过量,这对于大规模制备具有药学意义的手性化合物至关重要 (Bendigiri 等人,2018 年)。
反应性低聚物的合成:Morinaga 等人 (2014 年) 使用四正丁基氟化铵作为引发剂,对苯甲基缩水甘油醚 (GPE) 进行了无金属开环低聚合。这导致了具有受控分子量和官能团的反应性低聚物的生成,可用于各种化学应用中 (Morinaga 等人,2014 年)。
微乳液中的阴离子聚合:Maitre 等人 (2000 年) 探讨了苯基缩水甘油醚在微乳液中的阴离子聚合,产生了 α,ω-二羟基化的聚醚链。这项研究有助于理解微乳液中的离子聚合及其在材料科学中的潜在应用 (Maitre 等人,2000 年)。
嗜碱芽孢杆菌的生物催化:Bala 等人 (2010 年) 报道了使用嗜碱芽孢杆菌对芳基缩水甘油醚进行动力学拆分。他们的研究结果突出了使用微生物酶进行环氧化物对映选择性合成的潜力,这是药物生产中的一项重要工艺 (Bala 等人,2010 年)。
低聚化研究:Tanaka 等人 (1967 年) 研究了取代苯基缩水甘油醚的低聚化,深入了解了反应机理和这些低聚物在各种工业过程中的潜在应用 (Tanaka 等人,1967 年)。
作用机制
Target of Action
The primary target of ®-Glycidyl phenyl ether is enzymes involved in primary metabolic pathways . These enzymes play crucial roles in various biochemical reactions within the cell. The interaction of ®-Glycidyl phenyl ether with these enzymes can lead to significant changes in cellular processes .
Mode of Action
®-Glycidyl phenyl ether interacts with its targets primarily through a process known as acidic cleavage . During this process, the ether oxygen in the compound is protonated, forming a good leaving group. This group can then be eliminated as part of an S_N2, S_N1, or E1 reaction mechanism . The exact pathway is determined by the strong acid used and the type of substituents attached to the ether .
Biochemical Pathways
The interaction of ®-Glycidyl phenyl ether with its targets affects several biochemical pathways. One of the key pathways influenced is the phenylpropane pathway . This pathway is strongly enhanced under certain conditions, such as salt stress . The phenylpropane pathway is involved in the synthesis of essential oils, which are major secondary metabolites of aromatic plants .
Pharmacokinetics
Ethers in general have a small dipole moment due to the presence of an electronegative oxygen atom . This could potentially influence the compound’s bioavailability.
Result of Action
The molecular and cellular effects of ®-Glycidyl phenyl ether’s action are largely dependent on its interaction with its targets and the subsequent changes in biochemical pathways. For instance, the enhancement of the phenylpropane pathway could lead to an increase in the production of essential oils . Additionally, the compound’s interaction with enzymes could lead to significant changes in cellular processes .
Action Environment
Environmental factors can significantly influence the action, efficacy, and stability of ®-Glycidyl phenyl ether. Factors such as light, temperature, drought, salinity, heavy metals, seasonal variations, and mineral concentrations can have a significant impact on the chemical nature and stability of essential oil components . These factors can also regulate the biosynthesis and yield of essential oils .
安全和危害
未来方向
生化分析
Biochemical Properties
®-Glycidyl phenyl ether plays a significant role in biochemical reactions due to its ability to interact with various enzymes and proteins. One of the key interactions is with epoxide hydrolases, which catalyze the enantioselective ring opening of ®-glycidyl phenyl ether to produce enantiopure vicinal diols . This interaction is crucial for the synthesis of chiral drug intermediates. Additionally, ®-glycidyl phenyl ether can undergo anionic polymerization, further highlighting its versatility in biochemical processes .
Cellular Effects
®-Glycidyl phenyl ether has notable effects on various types of cells and cellular processes. It has been observed to influence cell signaling pathways, gene expression, and cellular metabolism. For instance, in Bacillus megaterium, ®-glycidyl phenyl ether showed high enantioselectivity and enhanced epoxide hydrolase activity when used with specific cosolvents . This indicates its potential impact on cellular functions and metabolic processes.
Molecular Mechanism
The molecular mechanism of ®-Glycidyl phenyl ether involves its interaction with biomolecules, leading to enzyme inhibition or activation and changes in gene expression. The compound’s epoxide group is highly reactive, allowing it to form covalent bonds with nucleophilic sites on enzymes and proteins. This can result in the inhibition of enzyme activity or the activation of specific biochemical pathways . Additionally, ®-glycidyl phenyl ether can induce changes in gene expression by interacting with transcription factors and other regulatory proteins.
Temporal Effects in Laboratory Settings
In laboratory settings, the effects of ®-Glycidyl phenyl ether can change over time due to its stability and degradation. The compound is known to be air-sensitive and should be stored under inert gas to maintain its stability . Over time, ®-glycidyl phenyl ether may degrade, leading to changes in its biochemical activity and long-term effects on cellular function. Studies have shown that prolonged exposure to ®-glycidyl phenyl ether can cause damage to organs through repeated exposure .
Dosage Effects in Animal Models
The effects of ®-Glycidyl phenyl ether vary with different dosages in animal models. At lower doses, the compound may exhibit beneficial effects, such as enhanced enzyme activity and improved metabolic processes. At higher doses, ®-glycidyl phenyl ether can cause toxic or adverse effects, including skin irritation, respiratory irritation, and potential damage to reproductive organs . It is important to carefully control the dosage to avoid these adverse effects.
Metabolic Pathways
®-Glycidyl phenyl ether is involved in various metabolic pathways, interacting with enzymes and cofactors that facilitate its conversion into other metabolites. The compound can undergo enzymatic hydrolysis by epoxide hydrolases, resulting in the formation of diols . These diols can then enter further metabolic pathways, contributing to the overall metabolic flux and affecting metabolite levels in cells.
Transport and Distribution
Within cells and tissues, ®-Glycidyl phenyl ether is transported and distributed through interactions with transporters and binding proteins. The compound’s lipophilic nature allows it to easily cross cell membranes and accumulate in specific cellular compartments . This distribution can influence its localization and accumulation, affecting its biochemical activity and interactions with other biomolecules.
Subcellular Localization
The subcellular localization of ®-Glycidyl phenyl ether is influenced by targeting signals and post-translational modifications that direct it to specific compartments or organelles. The compound has been observed to localize in the endoplasmic reticulum and other membrane-bound organelles, where it can interact with enzymes and proteins involved in various biochemical processes . This localization is crucial for its activity and function within cells.
属性
IUPAC Name |
(2R)-2-(phenoxymethyl)oxirane | |
---|---|---|
Source | PubChem | |
URL | https://pubchem.ncbi.nlm.nih.gov | |
Description | Data deposited in or computed by PubChem | |
InChI |
InChI=1S/C9H10O2/c1-2-4-8(5-3-1)10-6-9-7-11-9/h1-5,9H,6-7H2/t9-/m0/s1 | |
Source | PubChem | |
URL | https://pubchem.ncbi.nlm.nih.gov | |
Description | Data deposited in or computed by PubChem | |
InChI Key |
FQYUMYWMJTYZTK-VIFPVBQESA-N | |
Source | PubChem | |
URL | https://pubchem.ncbi.nlm.nih.gov | |
Description | Data deposited in or computed by PubChem | |
Canonical SMILES |
C1C(O1)COC2=CC=CC=C2 | |
Source | PubChem | |
URL | https://pubchem.ncbi.nlm.nih.gov | |
Description | Data deposited in or computed by PubChem | |
Isomeric SMILES |
C1[C@@H](O1)COC2=CC=CC=C2 | |
Source | PubChem | |
URL | https://pubchem.ncbi.nlm.nih.gov | |
Description | Data deposited in or computed by PubChem | |
Molecular Formula |
C9H10O2 | |
Source | PubChem | |
URL | https://pubchem.ncbi.nlm.nih.gov | |
Description | Data deposited in or computed by PubChem | |
DSSTOX Substance ID |
DTXSID901308155 | |
Record name | (2R)-2-(Phenoxymethyl)oxirane | |
Source | EPA DSSTox | |
URL | https://comptox.epa.gov/dashboard/DTXSID901308155 | |
Description | DSSTox provides a high quality public chemistry resource for supporting improved predictive toxicology. | |
Molecular Weight |
150.17 g/mol | |
Source | PubChem | |
URL | https://pubchem.ncbi.nlm.nih.gov | |
Description | Data deposited in or computed by PubChem | |
CAS RN |
71031-02-2 | |
Record name | (2R)-2-(Phenoxymethyl)oxirane | |
Source | CAS Common Chemistry | |
URL | https://commonchemistry.cas.org/detail?cas_rn=71031-02-2 | |
Description | CAS Common Chemistry is an open community resource for accessing chemical information. Nearly 500,000 chemical substances from CAS REGISTRY cover areas of community interest, including common and frequently regulated chemicals, and those relevant to high school and undergraduate chemistry classes. This chemical information, curated by our expert scientists, is provided in alignment with our mission as a division of the American Chemical Society. | |
Explanation | The data from CAS Common Chemistry is provided under a CC-BY-NC 4.0 license, unless otherwise stated. | |
Record name | (2R)-2-(Phenoxymethyl)oxirane | |
Source | EPA DSSTox | |
URL | https://comptox.epa.gov/dashboard/DTXSID901308155 | |
Description | DSSTox provides a high quality public chemistry resource for supporting improved predictive toxicology. | |
Synthesis routes and methods I
Procedure details
Synthesis routes and methods II
Procedure details
Synthesis routes and methods III
Procedure details
Synthesis routes and methods IV
Procedure details
Retrosynthesis Analysis
AI-Powered Synthesis Planning: Our tool employs the Template_relevance Pistachio, Template_relevance Bkms_metabolic, Template_relevance Pistachio_ringbreaker, Template_relevance Reaxys, Template_relevance Reaxys_biocatalysis model, leveraging a vast database of chemical reactions to predict feasible synthetic routes.
One-Step Synthesis Focus: Specifically designed for one-step synthesis, it provides concise and direct routes for your target compounds, streamlining the synthesis process.
Accurate Predictions: Utilizing the extensive PISTACHIO, BKMS_METABOLIC, PISTACHIO_RINGBREAKER, REAXYS, REAXYS_BIOCATALYSIS database, our tool offers high-accuracy predictions, reflecting the latest in chemical research and data.
Strategy Settings
Precursor scoring | Relevance Heuristic |
---|---|
Min. plausibility | 0.01 |
Model | Template_relevance |
Template Set | Pistachio/Bkms_metabolic/Pistachio_ringbreaker/Reaxys/Reaxys_biocatalysis |
Top-N result to add to graph | 6 |
Feasible Synthetic Routes
Q & A
Q1: How does the addition of Tween-80 affect the enzymatic activity of Bacillus megaterium epoxide hydrolase towards (R)-Glycidyl phenyl ether?
A1: The research paper demonstrates that the presence of Tween-80, a nonionic surfactant, significantly enhances the activity of Bacillus megaterium epoxide hydrolase towards (R)-Glycidyl phenyl ether []. This enhancement manifests as a nearly two-fold increase in the enzyme's apparent activity compared to when DMSO is used as a co-solvent. The researchers suggest that this improvement likely stems from Tween-80's ability to create an emulsion, improving substrate availability to the enzyme. Additionally, Tween-80 positively influences the enzyme's thermostability, increasing its half-life at 50°C by 2.5 times [].
体外研究产品的免责声明和信息
请注意,BenchChem 上展示的所有文章和产品信息仅供信息参考。 BenchChem 上可购买的产品专为体外研究设计,这些研究在生物体外进行。体外研究,源自拉丁语 "in glass",涉及在受控实验室环境中使用细胞或组织进行的实验。重要的是要注意,这些产品没有被归类为药物或药品,他们没有得到 FDA 的批准,用于预防、治疗或治愈任何医疗状况、疾病或疾病。我们必须强调,将这些产品以任何形式引入人类或动物的身体都是法律严格禁止的。遵守这些指南对确保研究和实验的法律和道德标准的符合性至关重要。