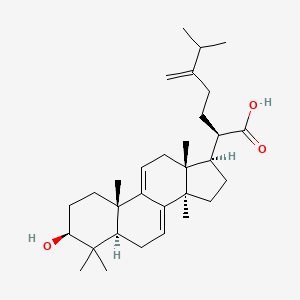
Dehydroeburicoic acid
Overview
Description
Dehydrotrametenolic acid is a lanostane-type triterpene acid isolated from the sclerotium of Poria cocos, a fungus commonly used in traditional Chinese medicine . This compound has garnered significant interest due to its diverse biological activities, including anti-inflammatory, anti-tumor, and anti-diabetic effects .
Preparation Methods
Synthetic Routes and Reaction Conditions: Dehydrotrametenolic acid is typically isolated from natural sources rather than synthesized chemically. The primary source is the sclerotium of Poria cocos . The extraction process involves drying the sclerotia, followed by solvent extraction and purification using chromatographic techniques .
Industrial Production Methods: Industrial production of dehydrotrametenolic acid follows similar extraction and purification methods as those used in laboratory settings. The large-scale extraction involves the use of organic solvents and advanced chromatographic techniques to ensure high purity and yield .
Chemical Reactions Analysis
Types of Reactions: Dehydrotrametenolic acid undergoes various chemical reactions, including oxidation, reduction, and substitution reactions .
Common Reagents and Conditions:
Oxidation: Common oxidizing agents such as potassium permanganate or chromium trioxide can be used to oxidize dehydrotrametenolic acid.
Reduction: Reducing agents like lithium aluminum hydride or sodium borohydride are employed to reduce the compound.
Substitution: Halogenation reactions can be carried out using reagents like bromine or chlorine under controlled conditions.
Major Products: The major products formed from these reactions include various oxidized, reduced, and halogenated derivatives of dehydrotrametenolic acid .
Scientific Research Applications
Antioxidant and Anti-inflammatory Properties
Dehydroeburicoic acid exhibits significant antioxidant and anti-inflammatory effects, making it a candidate for treating oxidative stress-related diseases. Research indicates that it acts as a dual inhibitor of the Keap1-Nrf2 protein-protein interaction and GSK3β, promoting Nrf2 nuclear translocation and the activation of downstream antioxidant genes. This mechanism is particularly relevant in models of alcoholic liver disease, where it restores mitochondrial function and exhibits minimal toxicity .
Antidiabetic Effects
The compound has shown promise in managing diabetes, particularly type 1 and type 2 diabetes. In animal studies, this compound improved glucose and lipid homeostasis, significantly reducing hyperglycemia and hyperlipidemia in high-fat diet-induced diabetic mice. It enhances the expression of glucose transporter 4 (GLUT4) and activates AMP-activated protein kinase (AMPK), which plays a crucial role in glucose metabolism .
Antitumor Activity
This compound has demonstrated antitumor properties against various cancer cell lines, notably human glioblastoma U87MG cells. Studies reveal that it induces necrosis through calcium- and calpain-dependent pathways, suggesting its potential as an anticancer agent . The compound's ability to inhibit tumor growth while sparing normal cells highlights its therapeutic potential.
Hepatoprotective Effects
The hepatoprotective effects of this compound have been documented in several studies. It protects liver cells from oxidative damage induced by carbon tetrachloride (CCl4) and alcohol, making it a candidate for liver disease therapies . Its protective mechanisms include modulating inflammatory responses and enhancing the liver's antioxidant capacity.
Interaction with Human Serum Albumin
Understanding the pharmacodynamics of this compound involves studying its interaction with human serum albumin (HSA). Fluorescence spectroscopy studies indicate that this compound binds to HSA primarily at site II, affecting drug transport and bioavailability. The binding parameters suggest that this interaction is crucial for the compound's pharmacokinetics .
Case Studies Overview
Mechanism of Action
Dehydrotrametenolic acid exerts its effects through several molecular pathways:
Apoptosis Induction: It induces apoptosis via the caspase-3 pathway, leading to programmed cell death in cancer cells.
Insulin Sensitization: The compound acts as an insulin sensitizer by activating peroxisome proliferator-activated receptor gamma (PPARγ), which plays a crucial role in glucose metabolism.
Anti-inflammatory Effects: It modulates the MAPK/AP-1 and IκBα/NF-κB pathways, reducing inflammation and promoting skin barrier function.
Comparison with Similar Compounds
Dehydrotrametenolic acid is compared with other lanostane-type triterpene acids, such as:
Pachymic Acid: Similar to dehydrotrametenolic acid, pachymic acid is also isolated from Poria cocos and exhibits anti-inflammatory and anti-tumor activities.
Tumulosic Acid: Another triterpene acid from Poria cocos, known for its anti-inflammatory properties.
Eburicoic Acid: Found in various fungi, this compound shares similar biological activities with dehydrotrametenolic acid.
Dehydrotrametenolic acid stands out due to its potent insulin-sensitizing effects and its ability to induce apoptosis through the caspase-3 pathway .
Biological Activity
Dehydroeburicoic acid (DEA), a triterpene derived from various medicinal fungi, particularly Antrodia camphorata and Taiwanofungus camphoratus, has garnered attention for its diverse biological activities. This article outlines the key findings regarding its biological activity, focusing on its antioxidant, anti-inflammatory, and antidiabetic properties.
Chemical Structure and Interaction
DEA is known for its unique molecular structure, which allows it to interact effectively with biological macromolecules. Studies utilizing fluorescence spectroscopy and molecular docking have demonstrated that DEA binds to human serum albumin (HSA), a critical transport protein in the bloodstream. The binding occurs primarily at site II of HSA, with significant interactions mediated by hydrogen bonds and hydrophobic forces. The binding constant and thermodynamic parameters indicate a stable interaction under physiological conditions, which is crucial for its pharmacokinetic properties .
Antioxidant Activity
One of the most significant biological activities of DEA is its role as an antioxidant. Research has shown that DEA acts as a dual inhibitor of the Keap1-Nrf2 protein-protein interaction (PPI) and glycogen synthase kinase 3 beta (GSK3β). This dual inhibition leads to increased nuclear translocation of Nrf2, which subsequently activates the transcription of antioxidant genes such as NQO1 and HO-1. In vitro studies demonstrated that DEA significantly enhances the expression of these genes in cells subjected to oxidative stress, outperforming other known inhibitors like ML334 .
Table 1: Antioxidant Gene Expression Induced by DEA
Gene | Expression Level (Fold Increase) |
---|---|
NQO1 | 2.0 |
HO-1 | 2.5 |
SOD2 | 2.0 |
Anti-Inflammatory Effects
DEA exhibits notable anti-inflammatory properties, particularly in models of liver injury induced by carbon tetrachloride (CCl4). It has been shown to reduce oxidative stress and tissue damage in liver cells, promoting recovery from inflammation . Additionally, studies on RAW 264.7 macrophage cells indicated that DEA can inhibit pro-inflammatory cytokine production, further supporting its potential as an anti-inflammatory agent .
Antidiabetic Activity
The antidiabetic effects of DEA have been extensively studied, particularly in high-fat diet-induced diabetic mouse models. DEA treatment resulted in significant improvements in glucose metabolism and lipid profiles. It enhanced the expression of glucose transporter 4 (GLUT4) and activated AMP-activated protein kinase (AMPK), leading to improved insulin sensitivity and reduced blood glucose levels .
Table 2: Effects of DEA on Metabolic Parameters in Diabetic Mice
Parameter | Control Group | DEA Treatment |
---|---|---|
Blood Glucose (mg/dL) | 200 | 120 |
Triglycerides (mg/dL) | 150 | 80 |
Insulin Levels (μU/mL) | 25 | 10 |
The mechanisms underlying the biological activities of DEA include:
- Nrf2 Activation : By inhibiting Keap1, DEA promotes Nrf2 nuclear translocation, enhancing the expression of various antioxidant genes.
- GSK3β Inhibition : The inhibition of GSK3β not only aids in Nrf2 activation but also contributes to improved metabolic functions.
- Modulation of Lipid Metabolism : DEA influences lipid metabolism by enhancing fatty acid oxidation and reducing lipogenesis in hepatic tissues.
Study on Nonalcoholic Fatty Liver Disease (NAFLD)
A recent study demonstrated that DEA significantly reduced lipid accumulation in liver tissues of mice fed a methionine-choline-deficient (MCD) diet. The compound upregulated aldehyde dehydrogenase 2 (ALDH2) activity, which plays a crucial role in detoxifying harmful aldehydes produced during lipid metabolism .
Study on Diabetes Management
In another study involving high-fat diet-induced diabetic mice, treatment with DEA led to a marked reduction in hyperglycemia and improved lipid profiles comparable to standard antidiabetic medications such as metformin .
Q & A
Basic Research Questions
Q. What experimental models are commonly used to study the antidiabetic effects of Dehydroeburicoic acid?
this compound (DHEA) is typically evaluated in high-fat-diet (HFD)-induced diabetic mouse models. For example, C57BL/6J mice fed an HFD for 8–12 weeks exhibit insulin resistance, hyperglycemia, and dyslipidemia. DHEA administration (e.g., 10–40 mg/kg/day) reduces blood glucose by activating AMP-activated protein kinase (AMPK) in skeletal muscle and liver, upregulating glucose transporter 4 (GLUT4), and enhancing fatty acid oxidation via peroxisome proliferator-activated receptor alpha (PPARα) .
Q. What are the standard protocols for isolating this compound from fungal sources?
DHEA is primarily extracted from Antrodia camphorata and Poria cocos using methanol or ethanol-based solvent extraction. The crude extract is purified via column chromatography (e.g., silica gel, Sephadex LH-20) and verified using HPLC with UV detection (λ = 254 nm). Structural confirmation is achieved through NMR spectroscopy (¹H, ¹³C) and mass spectrometry .
Q. How is the anticancer activity of this compound assessed in vitro?
Anticancer effects are evaluated using human glioblastoma U87MG cells. Key assays include:
- MTT assay : Measures cell viability via mitochondrial activity.
- LDH release assay : Quantifies necrotic cell death.
- Annexin V/PI staining : Distinguishes apoptosis from necrosis. DHEA (IC₅₀ ~20–50 µM) induces cytoplasmic vacuolization, mitochondrial membrane depolarization, and calcium overload, leading to calpain-dependent necrosis rather than apoptosis .
Advanced Research Questions
Q. How does this compound interact with human serum albumin (HSA), and what are the implications for pharmacokinetics?
Fluorescence quenching and molecular docking studies reveal that DHEA binds to HSA’s Site II (subdomain IIIA) via hydrogen bonding (Glu-492, Lys-545), hydrophobic interactions, and van der Waals forces. The binding constant (K ≈ 10⁴ M⁻¹) suggests moderate affinity, but stability decreases at higher temperatures (>37°C), limiting its potential for systemic delivery .
Q. What mechanisms underlie conflicting reports on DHEA’s effects on lipid metabolism?
Discrepancies arise from tissue-specific actions:
- Liver : DHEA suppresses lipid synthesis (↓FAS, SREBP1c) and enhances fatty acid oxidation (↑PPARα, CPT1a).
- Adipose tissue : It increases lipid synthesis (↑PPARγ) but reduces lipid accumulation via AMPK-mediated lipolysis. Experimental variables like dosage, treatment duration, and model selection (e.g., HFD vs. streptozotocin-induced diabetes) further complicate interpretations .
Q. How can researchers differentiate DHEA-induced necrosis from apoptosis in cancer cells?
Key markers include:
- Necrosis : ATP depletion, LDH release, cytoplasmic vacuolization, and calpain activation (inhibited by BAPTA-AM).
- Apoptosis : Caspase-3 cleavage, phosphatidylserine externalization (Annexin V+), and sub-G1 DNA content. DHEA predominantly triggers necrosis in U87MG cells, as shown by the absence of caspase-3 activation and insensitivity to pan-caspase inhibitors .
Q. What are the best practices for studying DHEA’s protein-ligand interactions using fluorescence spectroscopy?
- Use synchronous fluorescence to monitor conformational changes in HSA’s tyrosine/tryptophan residues.
- Employ three-dimensional fluorescence to map binding-induced shifts in emission spectra.
- Validate results with molecular docking (e.g., AutoDock Vina) to predict binding sites and interaction energies .
Q. How does DHEA modulate insulin signaling pathways in skeletal muscle?
DHEA enhances insulin sensitivity by phosphorylating Akt (Ser473) and AMPK (Thr172), which synergistically promote GLUT4 translocation to the plasma membrane. This mechanism is confirmed via Western blotting and glucose uptake assays in L6 myotubes and HFD mouse muscle tissue .
Q. Methodological Notes
- Data Validation : Cross-validate in vitro findings with in vivo models to account for tissue complexity.
- Dose Optimization : Conduct dose-response studies (e.g., 10–100 µM in vitro, 10–40 mg/kg in vivo) to avoid off-target effects.
- Ethical Compliance : Follow institutional guidelines for animal studies, including IACUC approval for diabetic mouse models .
Properties
IUPAC Name |
(2R)-2-[(3S,5R,10S,13R,14R,17R)-3-hydroxy-4,4,10,13,14-pentamethyl-2,3,5,6,12,15,16,17-octahydro-1H-cyclopenta[a]phenanthren-17-yl]-6-methyl-5-methylideneheptanoic acid | |
---|---|---|
Source | PubChem | |
URL | https://pubchem.ncbi.nlm.nih.gov | |
Description | Data deposited in or computed by PubChem | |
InChI |
InChI=1S/C31H48O3/c1-19(2)20(3)9-10-21(27(33)34)22-13-17-31(8)24-11-12-25-28(4,5)26(32)15-16-29(25,6)23(24)14-18-30(22,31)7/h11,14,19,21-22,25-26,32H,3,9-10,12-13,15-18H2,1-2,4-8H3,(H,33,34)/t21-,22-,25+,26+,29-,30-,31+/m1/s1 | |
Source | PubChem | |
URL | https://pubchem.ncbi.nlm.nih.gov | |
Description | Data deposited in or computed by PubChem | |
InChI Key |
ONFPYGOMAADWAT-OXUZYLMNSA-N | |
Source | PubChem | |
URL | https://pubchem.ncbi.nlm.nih.gov | |
Description | Data deposited in or computed by PubChem | |
Canonical SMILES |
CC(C)C(=C)CCC(C1CCC2(C1(CC=C3C2=CCC4C3(CCC(C4(C)C)O)C)C)C)C(=O)O | |
Source | PubChem | |
URL | https://pubchem.ncbi.nlm.nih.gov | |
Description | Data deposited in or computed by PubChem | |
Isomeric SMILES |
CC(C)C(=C)CC[C@H]([C@H]1CC[C@@]2([C@@]1(CC=C3C2=CC[C@@H]4[C@@]3(CC[C@@H](C4(C)C)O)C)C)C)C(=O)O | |
Source | PubChem | |
URL | https://pubchem.ncbi.nlm.nih.gov | |
Description | Data deposited in or computed by PubChem | |
Molecular Formula |
C31H48O3 | |
Source | PubChem | |
URL | https://pubchem.ncbi.nlm.nih.gov | |
Description | Data deposited in or computed by PubChem | |
DSSTOX Substance ID |
DTXSID501346470 | |
Record name | Dehydroeburicoic acid | |
Source | EPA DSSTox | |
URL | https://comptox.epa.gov/dashboard/DTXSID501346470 | |
Description | DSSTox provides a high quality public chemistry resource for supporting improved predictive toxicology. | |
Molecular Weight |
468.7 g/mol | |
Source | PubChem | |
URL | https://pubchem.ncbi.nlm.nih.gov | |
Description | Data deposited in or computed by PubChem | |
CAS No. |
6879-05-6 | |
Record name | Dehydroeburicoic acid | |
Source | CAS Common Chemistry | |
URL | https://commonchemistry.cas.org/detail?cas_rn=6879-05-6 | |
Description | CAS Common Chemistry is an open community resource for accessing chemical information. Nearly 500,000 chemical substances from CAS REGISTRY cover areas of community interest, including common and frequently regulated chemicals, and those relevant to high school and undergraduate chemistry classes. This chemical information, curated by our expert scientists, is provided in alignment with our mission as a division of the American Chemical Society. | |
Explanation | The data from CAS Common Chemistry is provided under a CC-BY-NC 4.0 license, unless otherwise stated. | |
Record name | Dehydroeburicoic acid | |
Source | EPA DSSTox | |
URL | https://comptox.epa.gov/dashboard/DTXSID501346470 | |
Description | DSSTox provides a high quality public chemistry resource for supporting improved predictive toxicology. | |
Retrosynthesis Analysis
AI-Powered Synthesis Planning: Our tool employs the Template_relevance Pistachio, Template_relevance Bkms_metabolic, Template_relevance Pistachio_ringbreaker, Template_relevance Reaxys, Template_relevance Reaxys_biocatalysis model, leveraging a vast database of chemical reactions to predict feasible synthetic routes.
One-Step Synthesis Focus: Specifically designed for one-step synthesis, it provides concise and direct routes for your target compounds, streamlining the synthesis process.
Accurate Predictions: Utilizing the extensive PISTACHIO, BKMS_METABOLIC, PISTACHIO_RINGBREAKER, REAXYS, REAXYS_BIOCATALYSIS database, our tool offers high-accuracy predictions, reflecting the latest in chemical research and data.
Strategy Settings
Precursor scoring | Relevance Heuristic |
---|---|
Min. plausibility | 0.01 |
Model | Template_relevance |
Template Set | Pistachio/Bkms_metabolic/Pistachio_ringbreaker/Reaxys/Reaxys_biocatalysis |
Top-N result to add to graph | 6 |
Feasible Synthetic Routes
Disclaimer and Information on In-Vitro Research Products
Please be aware that all articles and product information presented on BenchChem are intended solely for informational purposes. The products available for purchase on BenchChem are specifically designed for in-vitro studies, which are conducted outside of living organisms. In-vitro studies, derived from the Latin term "in glass," involve experiments performed in controlled laboratory settings using cells or tissues. It is important to note that these products are not categorized as medicines or drugs, and they have not received approval from the FDA for the prevention, treatment, or cure of any medical condition, ailment, or disease. We must emphasize that any form of bodily introduction of these products into humans or animals is strictly prohibited by law. It is essential to adhere to these guidelines to ensure compliance with legal and ethical standards in research and experimentation.