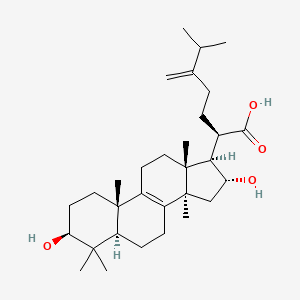
Tumulosic acid
- Click on QUICK INQUIRY to receive a quote from our team of experts.
- With the quality product at a COMPETITIVE price, you can focus more on your research.
Overview
Description
Tumulosic acid is a natural triterpenoid compound found in the fungus Wolfiporia cocos. It has a molecular formula of C31H50O4 and a molecular weight of 486.73 g/mol . This compound is known for its diverse biological activities and has been the subject of various scientific studies due to its potential therapeutic applications.
Scientific Research Applications
Chemistry: Used as a precursor for synthesizing other triterpenoid compounds.
Biology: Studied for its role in cellular processes and signaling pathways.
Medicine: Exhibits anticancer, anti-inflammatory, and antioxidant properties
Industry: Potential applications in the development of pharmaceuticals and nutraceuticals.
Mechanism of Action
Tumulosic acid has been found to have anti-proliferative activity against certain cancer cell lines . It is suggested that this compound might inhibit cancer cells by regulating BCL2L1, a protein that is highly expressed in certain types of cancer and is associated with a worse prognosis . It has also been found to induce cell apoptosis by modulating the PI3K/AKT signaling pathway .
Safety and Hazards
Preparation Methods
Synthetic Routes and Reaction Conditions: Tumulosic acid can be extracted from the roots of Wolfiporia cocos. The extraction process typically involves solvent extraction using solvents such as chloroform, dichloromethane, ethyl acetate, dimethyl sulfoxide, and acetone . The extracted compound is then purified using chromatographic techniques.
Industrial Production Methods: While there is limited information on the large-scale industrial production of this compound, the extraction from natural sources remains the primary method. Advances in biotechnological methods and synthetic biology may offer future avenues for more efficient production.
Chemical Reactions Analysis
Types of Reactions: Tumulosic acid undergoes various chemical reactions, including:
Oxidation: this compound can be oxidized to form dehydro-tumulosic acid.
Reduction: Reduction reactions can modify the functional groups present in this compound.
Substitution: Substitution reactions can introduce new functional groups into the this compound molecule.
Common Reagents and Conditions:
Oxidizing Agents: Common oxidizing agents include potassium permanganate and chromium trioxide.
Reducing Agents: Sodium borohydride and lithium aluminum hydride are commonly used reducing agents.
Substitution Reagents: Halogenating agents and nucleophiles are used for substitution reactions.
Major Products:
Dehydro-tumulosic acid: Formed through oxidation.
Various derivatives: Formed through substitution and reduction reactions.
Comparison with Similar Compounds
Tumulosic acid is part of a group of triterpenoid compounds found in Wolfiporia cocos. Similar compounds include:
- Dehydro-tumulosic acid
- Polyporenic acid C
- Pachymic acid
- Dehydrotrametenolic acid
- Dehydroeburicoic acid
Uniqueness: this compound stands out due to its specific biological activities and its potential as a therapeutic agent. Its ability to inhibit multiple enzymes and regulate key signaling pathways makes it a compound of significant interest in scientific research.
Properties
IUPAC Name |
(2R)-2-[(3S,5R,10S,13R,14R,16R,17R)-3,16-dihydroxy-4,4,10,13,14-pentamethyl-2,3,5,6,7,11,12,15,16,17-decahydro-1H-cyclopenta[a]phenanthren-17-yl]-6-methyl-5-methylideneheptanoic acid |
Source
|
---|---|---|
Source | PubChem | |
URL | https://pubchem.ncbi.nlm.nih.gov | |
Description | Data deposited in or computed by PubChem | |
InChI |
InChI=1S/C31H50O4/c1-18(2)19(3)9-10-20(27(34)35)26-23(32)17-31(8)22-11-12-24-28(4,5)25(33)14-15-29(24,6)21(22)13-16-30(26,31)7/h18,20,23-26,32-33H,3,9-17H2,1-2,4-8H3,(H,34,35)/t20-,23-,24+,25+,26+,29-,30-,31+/m1/s1 |
Source
|
Source | PubChem | |
URL | https://pubchem.ncbi.nlm.nih.gov | |
Description | Data deposited in or computed by PubChem | |
InChI Key |
XADJANKGURNTIA-YEXRKOARSA-N |
Source
|
Source | PubChem | |
URL | https://pubchem.ncbi.nlm.nih.gov | |
Description | Data deposited in or computed by PubChem | |
Canonical SMILES |
CC(C)C(=C)CCC(C1C(CC2(C1(CCC3=C2CCC4C3(CCC(C4(C)C)O)C)C)C)O)C(=O)O |
Source
|
Source | PubChem | |
URL | https://pubchem.ncbi.nlm.nih.gov | |
Description | Data deposited in or computed by PubChem | |
Isomeric SMILES |
CC(C)C(=C)CC[C@H]([C@H]1[C@@H](C[C@@]2([C@@]1(CCC3=C2CC[C@@H]4[C@@]3(CC[C@@H](C4(C)C)O)C)C)C)O)C(=O)O |
Source
|
Source | PubChem | |
URL | https://pubchem.ncbi.nlm.nih.gov | |
Description | Data deposited in or computed by PubChem | |
Molecular Formula |
C31H50O4 |
Source
|
Source | PubChem | |
URL | https://pubchem.ncbi.nlm.nih.gov | |
Description | Data deposited in or computed by PubChem | |
Molecular Weight |
486.7 g/mol |
Source
|
Source | PubChem | |
URL | https://pubchem.ncbi.nlm.nih.gov | |
Description | Data deposited in or computed by PubChem | |
Disclaimer and Information on In-Vitro Research Products
Please be aware that all articles and product information presented on BenchChem are intended solely for informational purposes. The products available for purchase on BenchChem are specifically designed for in-vitro studies, which are conducted outside of living organisms. In-vitro studies, derived from the Latin term "in glass," involve experiments performed in controlled laboratory settings using cells or tissues. It is important to note that these products are not categorized as medicines or drugs, and they have not received approval from the FDA for the prevention, treatment, or cure of any medical condition, ailment, or disease. We must emphasize that any form of bodily introduction of these products into humans or animals is strictly prohibited by law. It is essential to adhere to these guidelines to ensure compliance with legal and ethical standards in research and experimentation.