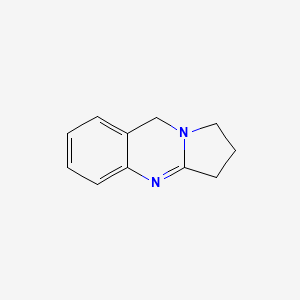
Deoxypeganine
Overview
Description
Mechanism of Action
Target of Action
Deoxypeganine, also known as Desoxypeganine, is an alkaloid that primarily targets cholinesterase (BChE and AChE) and monoamine oxidase-A (MAO-A) . Cholinesterases are key enzymes involved in the breakdown of acetylcholine, a neurotransmitter crucial for memory and cognition. Monoamine oxidase-A is an enzyme that degrades serotonin, norepinephrine, and dopamine, neurotransmitters that play significant roles in mood regulation .
Mode of Action
This compound acts as a potent inhibitor of both cholinesterase and selective MAO-A . By inhibiting these enzymes, it prevents the breakdown of their respective neurotransmitters, leading to an increase in the levels of these neurotransmitters in the brain . This can result in enhanced cognitive function and mood regulation .
Biochemical Pathways
The primary biochemical pathways affected by this compound are the cholinergic and monoaminergic pathways . By inhibiting the breakdown of acetylcholine and monoamines, this compound can potentially enhance the signaling in these pathways, leading to improved cognitive function and mood regulation .
Pharmacokinetics
This suggests that it is well-absorbed in the gastrointestinal tract and can reach its target sites in the body effectively .
Result of Action
The primary result of this compound’s action is the potential enhancement of cognitive function and mood regulation . By increasing the levels of acetylcholine and monoamines in the brain, it may help improve memory, cognition, and mood . It has been suggested for use in alcohol abuse research due to its potential effects on reducing alcohol intake and preference .
Action Environment
The action of this compound can be influenced by various environmental factors. For instance, light has been found to affect the stability of this compound hydrochloride . Additionally, the presence of other compounds, such as deoxyvasicinone hydrochloride, can influence the oxidation kinetics of this compound hydrochloride . These factors can potentially impact the efficacy and stability of this compound.
Biochemical Analysis
Biochemical Properties
Deoxypeganine plays a significant role in biochemical reactions by interacting with various enzymes and proteins. It is a potent inhibitor of cholinesterase enzymes, specifically butyrylcholinesterase and acetylcholinesterase, with IC50 values of 2 μM and 17 μM, respectively . Additionally, this compound selectively inhibits monoamine oxidase A with an IC50 value of 2 μM . These interactions suggest that this compound can modulate neurotransmitter levels in the brain, potentially offering therapeutic benefits for conditions such as Alzheimer’s disease and depression.
Cellular Effects
This compound exerts various effects on different cell types and cellular processes. In neuronal cells, this compound has been shown to reduce ethanol intake and preference in alcohol-preferring rats, indicating its potential use in treating alcohol dependence . The compound influences cell signaling pathways by inhibiting cholinesterase and monoamine oxidase enzymes, leading to increased levels of acetylcholine and monoamines, respectively. This modulation of neurotransmitter levels can impact gene expression and cellular metabolism, contributing to its neuroprotective effects.
Molecular Mechanism
The molecular mechanism of this compound involves its binding interactions with cholinesterase and monoamine oxidase enzymes. By inhibiting these enzymes, this compound prevents the breakdown of acetylcholine and monoamines, resulting in elevated levels of these neurotransmitters in the synaptic cleft . This inhibition can enhance cholinergic and monoaminergic signaling, which may contribute to its therapeutic effects in neurodegenerative diseases and mood disorders. Additionally, this compound’s interaction with these enzymes may lead to changes in gene expression related to neurotransmitter synthesis and degradation.
Temporal Effects in Laboratory Settings
In laboratory settings, the effects of this compound have been observed to change over time. Studies have shown that this compound is stable under standard laboratory conditions, with minimal degradation over extended periods . Long-term exposure to this compound in in vitro and in vivo studies has demonstrated sustained inhibition of cholinesterase and monoamine oxidase enzymes, leading to prolonged increases in neurotransmitter levels . These findings suggest that this compound can maintain its biochemical activity over time, making it a viable candidate for therapeutic applications.
Dosage Effects in Animal Models
The effects of this compound vary with different dosages in animal models. In studies involving alcohol-preferring rats, this compound was administered at doses of 10, 20, and 30 mg/kg, resulting in a dose-dependent reduction in ethanol intake and preference . Higher doses of this compound have been associated with increased efficacy in reducing alcohol consumption, but potential toxic or adverse effects at very high doses have not been extensively studied. These findings highlight the importance of determining optimal dosages for therapeutic use while minimizing potential side effects.
Metabolic Pathways
This compound is involved in several metabolic pathways, interacting with enzymes and cofactors that influence its metabolism. The primary metabolic pathway of this compound involves its oxidation to form deoxyvasicinone and other metabolites . These metabolites are further processed by various enzymes, including cytochrome P450 enzymes, which play a crucial role in the compound’s biotransformation . The metabolic flux and levels of metabolites can be influenced by factors such as enzyme activity and the presence of cofactors, affecting the overall pharmacokinetics of this compound.
Transport and Distribution
This compound is transported and distributed within cells and tissues through various mechanisms. The compound can cross the blood-brain barrier, allowing it to exert its effects on the central nervous system . Within cells, this compound may interact with transporters and binding proteins that facilitate its uptake and distribution. These interactions can influence the localization and accumulation of this compound in specific tissues, affecting its overall pharmacological activity.
Subcellular Localization
The subcellular localization of this compound is critical for its activity and function. This compound is primarily localized in the cytoplasm, where it interacts with cholinesterase and monoamine oxidase enzymes . The compound may also be directed to specific subcellular compartments through targeting signals or post-translational modifications. These localization mechanisms ensure that this compound can effectively inhibit its target enzymes and exert its biochemical effects within the cell.
Preparation Methods
Synthetic Routes and Reaction Conditions
Desoxypeganine can be synthesized through various chemical routes. One common method involves the extraction of the compound from the seeds of Peganum harmala using organic solvents. The extracted material is then subjected to purification processes such as column chromatography to isolate desoxypeganine .
Industrial Production Methods
Industrial production of desoxypeganine typically involves large-scale extraction from plant sources. The seeds of Peganum harmala are harvested and processed to extract the alkaloid. The extraction process involves the use of solvents like ethanol or methanol, followed by purification steps to obtain the pure compound .
Chemical Reactions Analysis
Types of Reactions
Desoxypeganine undergoes various chemical reactions, including:
Oxidation: Desoxypeganine can be oxidized to form corresponding quinoline derivatives.
Reduction: Reduction reactions can convert desoxypeganine to its reduced forms.
Substitution: Substitution reactions can occur at various positions on the quinoline ring.
Common Reagents and Conditions
Oxidation: Common oxidizing agents include potassium permanganate and hydrogen peroxide.
Reduction: Reducing agents such as sodium borohydride and lithium aluminum hydride are used.
Substitution: Reagents like halogens and alkylating agents are employed for substitution reactions.
Major Products Formed
The major products formed from these reactions include various quinoline derivatives, which can have different pharmacological properties .
Scientific Research Applications
Desoxypeganine has several scientific research applications:
Chemistry: Used as a model compound for studying alkaloid chemistry and reaction mechanisms.
Biology: Investigated for its effects on cholinesterase enzymes and monoamine oxidase A, making it a valuable tool in neurochemical research.
Medicine: Explored for its potential in treating alcohol abuse and as a smoking cessation aid due to its inhibitory effects on cholinesterase and monoamine oxidase A
Industry: Utilized in the development of pharmacological agents targeting neurological disorders.
Comparison with Similar Compounds
Similar Compounds
Vasicine: Another alkaloid found in Peganum harmala, known for its bronchodilator and respiratory stimulant properties.
Galanthamine: An alkaloid used in the treatment of Alzheimer’s disease due to its cholinesterase inhibitory effects.
Physostigmine: A cholinesterase inhibitor used to treat glaucoma and myasthenia gravis.
Uniqueness of Desoxypeganine
Desoxypeganine is unique due to its dual inhibitory effects on both cholinesterase enzymes and monoamine oxidase A. This dual action makes it a promising candidate for treating neurological disorders and substance abuse, setting it apart from other similar compounds .
Properties
IUPAC Name |
1,2,3,9-tetrahydropyrrolo[2,1-b]quinazoline | |
---|---|---|
Source | PubChem | |
URL | https://pubchem.ncbi.nlm.nih.gov | |
Description | Data deposited in or computed by PubChem | |
InChI |
InChI=1S/C11H12N2/c1-2-5-10-9(4-1)8-13-7-3-6-11(13)12-10/h1-2,4-5H,3,6-8H2 | |
Source | PubChem | |
URL | https://pubchem.ncbi.nlm.nih.gov | |
Description | Data deposited in or computed by PubChem | |
InChI Key |
WUFQLZTXIWKION-UHFFFAOYSA-N | |
Source | PubChem | |
URL | https://pubchem.ncbi.nlm.nih.gov | |
Description | Data deposited in or computed by PubChem | |
Canonical SMILES |
C1CC2=NC3=CC=CC=C3CN2C1 | |
Source | PubChem | |
URL | https://pubchem.ncbi.nlm.nih.gov | |
Description | Data deposited in or computed by PubChem | |
Molecular Formula |
C11H12N2 | |
Source | PubChem | |
URL | https://pubchem.ncbi.nlm.nih.gov | |
Description | Data deposited in or computed by PubChem | |
Related CAS |
61939-05-7 (hydrochloride) | |
Record name | 3-Deoxyvasicine | |
Source | ChemIDplus | |
URL | https://pubchem.ncbi.nlm.nih.gov/substance/?source=chemidplus&sourceid=0000495590 | |
Description | ChemIDplus is a free, web search system that provides access to the structure and nomenclature authority files used for the identification of chemical substances cited in National Library of Medicine (NLM) databases, including the TOXNET system. | |
DSSTOX Substance ID |
DTXSID40197807 | |
Record name | 3-Deoxyvasicine | |
Source | EPA DSSTox | |
URL | https://comptox.epa.gov/dashboard/DTXSID40197807 | |
Description | DSSTox provides a high quality public chemistry resource for supporting improved predictive toxicology. | |
Molecular Weight |
172.23 g/mol | |
Source | PubChem | |
URL | https://pubchem.ncbi.nlm.nih.gov | |
Description | Data deposited in or computed by PubChem | |
CAS No. |
495-59-0 | |
Record name | Deoxypeganine | |
Source | CAS Common Chemistry | |
URL | https://commonchemistry.cas.org/detail?cas_rn=495-59-0 | |
Description | CAS Common Chemistry is an open community resource for accessing chemical information. Nearly 500,000 chemical substances from CAS REGISTRY cover areas of community interest, including common and frequently regulated chemicals, and those relevant to high school and undergraduate chemistry classes. This chemical information, curated by our expert scientists, is provided in alignment with our mission as a division of the American Chemical Society. | |
Explanation | The data from CAS Common Chemistry is provided under a CC-BY-NC 4.0 license, unless otherwise stated. | |
Record name | 3-Deoxyvasicine | |
Source | ChemIDplus | |
URL | https://pubchem.ncbi.nlm.nih.gov/substance/?source=chemidplus&sourceid=0000495590 | |
Description | ChemIDplus is a free, web search system that provides access to the structure and nomenclature authority files used for the identification of chemical substances cited in National Library of Medicine (NLM) databases, including the TOXNET system. | |
Record name | 3-Deoxyvasicine | |
Source | EPA DSSTox | |
URL | https://comptox.epa.gov/dashboard/DTXSID40197807 | |
Description | DSSTox provides a high quality public chemistry resource for supporting improved predictive toxicology. | |
Record name | DEOXYPEGANINE | |
Source | FDA Global Substance Registration System (GSRS) | |
URL | https://gsrs.ncats.nih.gov/ginas/app/beta/substances/A3P6YTL6RH | |
Description | The FDA Global Substance Registration System (GSRS) enables the efficient and accurate exchange of information on what substances are in regulated products. Instead of relying on names, which vary across regulatory domains, countries, and regions, the GSRS knowledge base makes it possible for substances to be defined by standardized, scientific descriptions. | |
Explanation | Unless otherwise noted, the contents of the FDA website (www.fda.gov), both text and graphics, are not copyrighted. They are in the public domain and may be republished, reprinted and otherwise used freely by anyone without the need to obtain permission from FDA. Credit to the U.S. Food and Drug Administration as the source is appreciated but not required. | |
Retrosynthesis Analysis
AI-Powered Synthesis Planning: Our tool employs the Template_relevance Pistachio, Template_relevance Bkms_metabolic, Template_relevance Pistachio_ringbreaker, Template_relevance Reaxys, Template_relevance Reaxys_biocatalysis model, leveraging a vast database of chemical reactions to predict feasible synthetic routes.
One-Step Synthesis Focus: Specifically designed for one-step synthesis, it provides concise and direct routes for your target compounds, streamlining the synthesis process.
Accurate Predictions: Utilizing the extensive PISTACHIO, BKMS_METABOLIC, PISTACHIO_RINGBREAKER, REAXYS, REAXYS_BIOCATALYSIS database, our tool offers high-accuracy predictions, reflecting the latest in chemical research and data.
Strategy Settings
Precursor scoring | Relevance Heuristic |
---|---|
Min. plausibility | 0.01 |
Model | Template_relevance |
Template Set | Pistachio/Bkms_metabolic/Pistachio_ringbreaker/Reaxys/Reaxys_biocatalysis |
Top-N result to add to graph | 6 |
Feasible Synthetic Routes
Q1: What is the primary mechanism of action of deoxypeganine?
A1: this compound acts as a reversible acetylcholinesterase inhibitor. [] This means it prevents the breakdown of acetylcholine, a neurotransmitter crucial for learning, memory, and muscle control.
Q2: What are the downstream effects of this compound's acetylcholinesterase inhibition?
A2: By inhibiting acetylcholinesterase, this compound increases acetylcholine levels in the brain. This can lead to improved cognitive function, particularly in conditions characterized by acetylcholine deficiency, such as Alzheimer's dementia. [, ]
Q3: What is the molecular formula and weight of this compound?
A3: Unfortunately, the provided research abstracts do not explicitly state the molecular formula and weight of this compound. For precise information, refer to comprehensive chemical databases or primary research articles focusing on the compound's structural elucidation.
Q4: Is there spectroscopic data available for this compound?
A4: While the abstracts mention techniques like Gas Chromatography-Mass Spectrometry (GC-MS) [] and Nuclear Magnetic Resonance (NMR) [] for analyzing this compound and related compounds, they do not provide detailed spectroscopic data. To access this information, consult research articles specifically dedicated to this compound's structural characterization.
Q5: How does this compound interact with other materials?
A5: Research indicates that this compound can form complexes with zinc chloride, facilitating its isolation from plant extracts. [] Additionally, studies exploring its incorporation into drug delivery systems demonstrate its compatibility with materials like silk fibroin, where it forms hydrogen bonds. []
Q6: What is known about the stability of this compound?
A6: While the provided abstracts lack specific details on this compound's stability under various conditions, research highlights the use of techniques like mechanochemical modification to potentially enhance its stability and properties. [, ] Further research is needed to fully understand its stability profile.
Q7: Does this compound exhibit any catalytic properties?
A7: The provided abstracts primarily focus on this compound's pharmacological properties and do not mention any catalytic activities.
Q8: Have computational methods been used to study this compound?
A8: Yes, quantum-mechanical investigations have been conducted to understand the reduction of this compound and its derivatives. [] Additionally, research has explored the photochemical oxidation of this compound using computational modeling. [, ]
Q9: How do structural modifications impact this compound's activity?
A9: Research on the neuroprotective activity of this compound derivatives revealed that two amino derivatives exhibited greater potency than this compound itself. [] This suggests that modifications to the this compound structure can influence its pharmacological activity and warrant further investigation.
Disclaimer and Information on In-Vitro Research Products
Please be aware that all articles and product information presented on BenchChem are intended solely for informational purposes. The products available for purchase on BenchChem are specifically designed for in-vitro studies, which are conducted outside of living organisms. In-vitro studies, derived from the Latin term "in glass," involve experiments performed in controlled laboratory settings using cells or tissues. It is important to note that these products are not categorized as medicines or drugs, and they have not received approval from the FDA for the prevention, treatment, or cure of any medical condition, ailment, or disease. We must emphasize that any form of bodily introduction of these products into humans or animals is strictly prohibited by law. It is essential to adhere to these guidelines to ensure compliance with legal and ethical standards in research and experimentation.