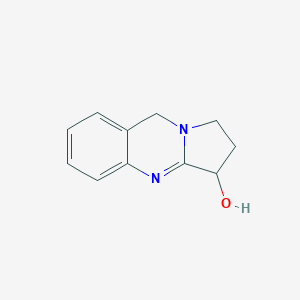
Vasicine
Overview
Description
Vasicine (C₁₁H₁₂N₂O), also known as peganine, is a quinazoline alkaloid predominantly isolated from Adhatoda vasica (Malabar nut) and Peganum harmala (Syrian rue) . It is a key bioactive compound in Ayurvedic and Unani medicine, recognized for its bronchodilatory, antioxidant, and anti-ulcer properties. Pharmacologically, this compound inhibits H⁺-K⁺-ATPase (IC₅₀: 73.47 µg/mL), demonstrating anti-secretory and cytoprotective effects in gastric ulcer models . Its derivatives, bromhexine and ambroxol, are clinically used as mucolytics and expectorants . Structural characterization via NMR, IR, and mass spectroscopy confirms its bicyclic quinazoline framework .
Preparation Methods
Synthetic Routes and Reaction Conditions: Vasicine can be synthesized through various methods, including high-performance thin-layer chromatography (HPTLC) and high-performance liquid chromatography (HPLC). One common method involves the extraction of this compound from the leaves of Justicia adhatoda using solvents like methanol, acetone, and acetonitrile. The extraction process can be enhanced using techniques such as ultrasonic-assisted extraction (UAE) and microwave-assisted extraction (MAE) .
Industrial Production Methods: Industrial production of this compound typically involves the extraction from plant sources. The leaves of Justicia adhatoda are dried and powdered, followed by extraction using solvents. The extract is then purified using column chromatography and preparative thin-layer chromatography to isolate this compound .
Chemical Reactions Analysis
Types of Reactions: Vasicine undergoes various chemical reactions, including oxidation, reduction, and substitution. It is known to form several metabolites through processes such as monohydroxylation, dihydroxylation, trihydroxylation, oxidation, desaturation, sulfation, and glucuronidation .
Common Reagents and Conditions:
Oxidation: this compound can be oxidized to form vasicinone using oxidizing agents like potassium permanganate.
Reduction: Reduction of this compound can be achieved using reducing agents such as sodium borohydride.
Substitution: Substitution reactions involving this compound often use reagents like halogens and acids.
Major Products Formed: The major products formed from these reactions include vasicinone, vasicinol, and vasicinolone .
Scientific Research Applications
Biological Activities
Vasicine exhibits a range of biological activities, making it a subject of interest in pharmacological research. Key applications include:
- Antimicrobial Activity : this compound has shown significant antimicrobial effects against various pathogens. In vitro studies have demonstrated its efficacy in inhibiting bacterial growth, as evidenced by colony-forming unit assays .
- Antioxidant Activity : The compound displays strong antioxidant properties, with IC50 values indicating its effectiveness in scavenging free radicals:
- Anti-inflammatory Activity : this compound has been tested for anti-inflammatory effects through various assays, showing potential in reducing inflammation markers:
- Antidiabetic Activity : The compound inhibits enzymes related to diabetes management:
- Antiviral Activity : this compound has demonstrated antiviral properties, particularly against HIV protease with an IC50 value of 38.5 µg/ml .
- Anticancer Activity : Research indicates this compound's potential in cancer treatment, particularly against lung cancer cells (IC50 = 46.5 µg/ml) and human fibroblast cells (IC50 = 82.5 µg/ml) .
Agricultural Applications
Recent studies have also explored the application of this compound in agriculture, particularly its effects on crop growth and yield:
- Impact on Tomato Growth : A study indicated that this compound enhances physiological traits and yield in tomato plants when combined with recommended dose fertilizers (RDF). The leaf area increased significantly compared to control plants at both growth stages (60 and 90 days) .
Data Table: Summary of Biological Activities
Activity Type | Assay Type | IC50 Value (µg/ml) |
---|---|---|
Antimicrobial | Colony-forming unit assay | Not specified |
Antioxidant | ABTS scavenging | 11.5 |
DPPH radical scavenging | 18.2 | |
Anti-inflammatory | Proteinase inhibitory | 76 |
BSA method | 51.7 | |
Antidiabetic | α-amylase inhibition | 47.6 |
α-glucosidase inhibition | 49.68 | |
Antiviral | HIV protease inhibition | 38.5 |
Anticancer | Lung cancer cells | 46.5 |
Case Study 1: Anticancer Potential
A study focused on the cytotoxicity of this compound against lung carcinoma cells utilized multiple assays, including MTT and direct microscopic observation, confirming its potential as an anticancer agent .
Case Study 2: Agricultural Enhancement
Research conducted on the impact of this compound on tomato plants revealed that its application significantly improved growth metrics and yield, suggesting its utility as a natural growth enhancer in agriculture .
Mechanism of Action
Vasicine exerts its effects through various mechanisms:
Bronchodilatory Action: this compound relaxes the bronchial muscles, thereby easing breathing in conditions like asthma.
Expectorant Action: It increases the production of serous mucus in the respiratory tract, making phlegm thinner and less viscous, which helps in clearing the airways.
Anti-inflammatory Action: this compound inhibits the production of pro-inflammatory cytokines, reducing inflammation in the respiratory tract.
Acetylcholinesterase Inhibition: this compound inhibits the enzyme acetylcholinesterase, which increases the levels of acetylcholine in the brain, potentially improving cognitive function.
Comparison with Similar Compounds
Comparison with Structurally and Functionally Similar Compounds
Vasicinone
- Source : Co-occurs with vasicine in A. vasica .
- Structure : Oxidized form of this compound, with a ketone group replacing the hydroxyl group.
- Activity: Shares bronchodilatory effects but exhibits weaker antioxidant capacity. In ferric reducing assays, this compound showed 62% inhibition at 100 µg/mL (IC₅₀: 15 µg/mL), while vasicinone data are less documented .
Bromhexine and Ambroxol
- Source : Synthetic derivatives of this compound .
- Structure : Bromhexine incorporates a brominated phenyl group; ambroxol is its active metabolite.
- Activity : Both enhance mucus clearance via stimulation of surfactant production. Unlike this compound, they lack direct antioxidant or anti-ulcer activity but have superior clinical efficacy in chronic respiratory diseases .
Harmaline and Harmine
- Source : β-carboline alkaloids from P. harmala .
- Structure : β-carboline core vs. This compound’s quinazoline.
- Activity: Primarily antitumor and psychoactive. Harmaline inhibits monoamine oxidase-A (MAO-A), whereas this compound targets H⁺-K⁺-ATPase, reflecting divergent mechanisms .
This compound Acetate
- Source : Semisynthetic derivative of this compound .
- Structure : Acetylated at the hydroxyl group.
- Activity : Enhanced antimicrobial potency (against Staphylococcus aureus and Escherichia coli) and antioxidant capacity compared to this compound. Exact IC₅₀ values remain unpublished .
(±)-Vasicine
- Source : Racemic mixture of this compound enantiomers .
- Activity : Retains H⁺-K⁺-ATPase inhibition (IC₅₀: 73.47 µg/mL) but may exhibit altered pharmacokinetics due to stereochemical differences .
Data Table: Comparative Analysis of this compound and Analogues
Key Research Findings
- Antioxidant Capacity : this compound’s ferric reducing power (62% inhibition at 100 µg/mL) surpasses many plant alkaloids but is inferior to ascorbic acid (82%) .
- Structural-Activity Relationship (SAR) : The hydroxyl group in this compound is critical for H⁺-K⁺-ATPase inhibition; acetylation (this compound acetate) shifts activity toward antimicrobial effects .
Biological Activity
Vasicine, a quinazoline alkaloid derived from the plant Adhatoda vasica, has garnered significant attention for its diverse biological activities. This article explores the various pharmacological effects of this compound, including its anticancer, anti-inflammatory, antioxidant, antidiabetic, and antiviral properties, supported by empirical data and case studies.
This compound is characterized by a quinazoline skeleton, which is crucial for its biological activities. This structural feature enables this compound to interact with various biological targets, influencing multiple signaling pathways. In silico studies have shown that this compound exhibits good binding affinity to key enzymes such as α-amylase and cyclooxygenase, suggesting potential therapeutic applications in metabolic and inflammatory disorders .
Anticancer Activity
This compound demonstrates promising anticancer properties. It has been reported to inhibit the growth of lung cancer cells with an IC50 value of 46.5 µg/ml and human fibroblast cells at 82.5 µg/ml. These findings indicate that this compound may serve as a potential chemotherapeutic agent against specific cancer types .
Anti-inflammatory Activity
The anti-inflammatory effects of this compound were evaluated using various assays:
- Proteinase Inhibitory Activity : this compound showed an IC50 of 76 µg/ml.
- Bovine Serum Albumin (BSA) Method : The IC50 was recorded at 51.7 µg/ml.
- Egg Albumin Method : An IC50 of 53.2 µg/ml was observed.
These results suggest that this compound effectively inhibits inflammation-related processes, potentially benefiting conditions like arthritis and other inflammatory diseases .
Antioxidant Activity
This compound exhibits significant antioxidant properties, as demonstrated by various assays:
Assay Type | IC50 (µg/ml) |
---|---|
ABTS Scavenging | 11.5 |
Ferric Reducing Power (FRAP) | 15.0 |
DPPH Radical Scavenging | 18.2 |
Hydroxyl Radical Scavenging | 22.0 |
Hydrogen Peroxide Assay | 27.8 |
These results indicate that this compound can scavenge free radicals effectively, which is essential in preventing oxidative stress-related diseases .
Antidiabetic Activity
This compound has shown antidiabetic properties through several in vitro assays:
- α-Amylase Inhibition : IC50 = 47.6 µg/ml
- α-Glucosidase Inhibition : IC50 = 49.68 µg/ml
These findings suggest that this compound may help manage blood glucose levels by inhibiting carbohydrate-digesting enzymes, thus providing a potential therapeutic avenue for diabetes management .
Antiviral Activity
Research indicates that this compound possesses antiviral activity against HIV protease with an IC50 value of 38.5 µg/ml. This suggests its potential utility in the treatment or management of viral infections .
Case Studies and Clinical Applications
A study involving neuroinflammatory models demonstrated that this compound significantly improved behavioral parameters and biochemical markers in zebrafish models subjected to neuroinflammation due to surgery or chemical exposure . This highlights its potential application in neurological disorders.
Q & A
Basic Research Questions
Q. What validated chromatographic methods are recommended for quantifying vasicine in plant extracts, and how are they optimized for accuracy?
- Methodological Answer: High-performance liquid chromatography (HPLC) with UV detection is widely used, employing C18 columns and mobile phases like methanol:water (70:30 v/v) at 1.0 mL/min flow rate. Validation parameters include linearity (R² >0.99), limit of detection (LOD: ~0.1 µg/mL), and recovery rates (95–105%) via spiked samples. Thin-layer chromatography (TLC) with Dragendorff’s reagent is a qualitative alternative .
Q. Which in vitro models are standard for evaluating this compound’s bronchodilatory effects, and what endpoints are measured?
- Methodological Answer: Isolated guinea pig tracheal chains or human bronchial smooth muscle cells are common. Contraction-relaxation assays use histamine-induced spasms, measuring tension changes via force transducers. Endpoints include EC₅₀ values and comparison with reference agents (e.g., salbutamol). Cell viability assays (MTT) ensure non-cytotoxic concentrations .
Q. What pharmacokinetic parameters are critical in preclinical studies of this compound?
- Methodological Answer: Key parameters include bioavailability (oral vs. intravenous administration), plasma half-life (t₁/₂), volume of distribution (Vd), and metabolic pathways (e.g., cytochrome P450 isoforms). LC-MS/MS is used for plasma analysis, with sampling at 0.5, 1, 2, 4, 8, 12, and 24 hours post-administration .
Advanced Research Questions
Q. How can researchers resolve discrepancies in reported cytotoxicity of this compound across different cancer cell lines?
- Methodological Answer:
- Variable Control: Standardize cell culture conditions (e.g., passage number, media composition).
- Dose-Response Validation: Use a wide concentration range (0.1–100 µM) and replicate across labs.
- Mechanistic Follow-Up: Combine apoptosis assays (Annexin V/PI staining) with transcriptomic profiling (RNA-seq) to identify cell-line-specific pathways.
- Reference: Conflicting data may arise from differential expression of drug transporters (e.g., ABCB1) or metabolic enzymes .
Q. What experimental strategies optimize this compound extraction yields while preserving structural integrity?
- Methodological Answer:
- Solvent Selection: Ethanol-water mixtures (60–80% ethanol) balance polarity for alkaloid solubility.
- Green Techniques: Ultrasound-assisted extraction (20 kHz, 30°C, 20 min) increases yield by 15–20% vs. maceration.
- Stability Monitoring: Post-extraction, store at -20°C under nitrogen to prevent oxidation. Confirm stability via NMR (absence of new peaks) over 6 months .
Q. How should researchers design studies to clarify this compound’s dual role as a bronchodilator and potential hepatotoxin?
- Methodological Answer:
- Dual-Arm Design:
- Arm 1: Bronchodilatory efficacy in ovalbumin-sensitized murine models (FEV₁ measurements).
- Arm 2: Hepatotoxicity screening via serum ALT/AST levels and liver histopathology.
- Dose Differentiation: Identify therapeutic index (TI) by comparing ED₅₀ (bronchodilation) with LD₅₀ (hepatotoxicity).
- Mechanistic Links: Use metabolomics to assess if hepatotoxicity correlates with reactive metabolite formation (e.g., glutathione depletion assays) .
Properties
IUPAC Name |
1,2,3,9-tetrahydropyrrolo[2,1-b]quinazolin-3-ol | |
---|---|---|
Source | PubChem | |
URL | https://pubchem.ncbi.nlm.nih.gov | |
Description | Data deposited in or computed by PubChem | |
InChI |
InChI=1S/C11H12N2O/c14-10-5-6-13-7-8-3-1-2-4-9(8)12-11(10)13/h1-4,10,14H,5-7H2 | |
Source | PubChem | |
URL | https://pubchem.ncbi.nlm.nih.gov | |
Description | Data deposited in or computed by PubChem | |
InChI Key |
YIICVSCAKJMMDJ-UHFFFAOYSA-N | |
Source | PubChem | |
URL | https://pubchem.ncbi.nlm.nih.gov | |
Description | Data deposited in or computed by PubChem | |
Canonical SMILES |
C1CN2CC3=CC=CC=C3N=C2C1O | |
Source | PubChem | |
URL | https://pubchem.ncbi.nlm.nih.gov | |
Description | Data deposited in or computed by PubChem | |
Molecular Formula |
C11H12N2O | |
Source | PubChem | |
URL | https://pubchem.ncbi.nlm.nih.gov | |
Description | Data deposited in or computed by PubChem | |
DSSTOX Substance ID |
DTXSID701317988 | |
Record name | (±)-Vasicine | |
Source | EPA DSSTox | |
URL | https://comptox.epa.gov/dashboard/DTXSID701317988 | |
Description | DSSTox provides a high quality public chemistry resource for supporting improved predictive toxicology. | |
Molecular Weight |
188.23 g/mol | |
Source | PubChem | |
URL | https://pubchem.ncbi.nlm.nih.gov | |
Description | Data deposited in or computed by PubChem | |
CAS No. |
6159-56-4, 6159-55-3 | |
Record name | (±)-Vasicine | |
Source | CAS Common Chemistry | |
URL | https://commonchemistry.cas.org/detail?cas_rn=6159-56-4 | |
Description | CAS Common Chemistry is an open community resource for accessing chemical information. Nearly 500,000 chemical substances from CAS REGISTRY cover areas of community interest, including common and frequently regulated chemicals, and those relevant to high school and undergraduate chemistry classes. This chemical information, curated by our expert scientists, is provided in alignment with our mission as a division of the American Chemical Society. | |
Explanation | The data from CAS Common Chemistry is provided under a CC-BY-NC 4.0 license, unless otherwise stated. | |
Record name | DL-Vasicine | |
Source | ChemIDplus | |
URL | https://pubchem.ncbi.nlm.nih.gov/substance/?source=chemidplus&sourceid=0006159564 | |
Description | ChemIDplus is a free, web search system that provides access to the structure and nomenclature authority files used for the identification of chemical substances cited in National Library of Medicine (NLM) databases, including the TOXNET system. | |
Record name | (±)-Vasicine | |
Source | EPA DSSTox | |
URL | https://comptox.epa.gov/dashboard/DTXSID701317988 | |
Description | DSSTox provides a high quality public chemistry resource for supporting improved predictive toxicology. | |
Record name | Vasicine | |
Source | European Chemicals Agency (ECHA) | |
URL | https://echa.europa.eu/substance-information/-/substanceinfo/100.025.619 | |
Description | The European Chemicals Agency (ECHA) is an agency of the European Union which is the driving force among regulatory authorities in implementing the EU's groundbreaking chemicals legislation for the benefit of human health and the environment as well as for innovation and competitiveness. | |
Explanation | Use of the information, documents and data from the ECHA website is subject to the terms and conditions of this Legal Notice, and subject to other binding limitations provided for under applicable law, the information, documents and data made available on the ECHA website may be reproduced, distributed and/or used, totally or in part, for non-commercial purposes provided that ECHA is acknowledged as the source: "Source: European Chemicals Agency, http://echa.europa.eu/". Such acknowledgement must be included in each copy of the material. ECHA permits and encourages organisations and individuals to create links to the ECHA website under the following cumulative conditions: Links can only be made to webpages that provide a link to the Legal Notice page. | |
Record name | VASICINE, (±)- | |
Source | FDA Global Substance Registration System (GSRS) | |
URL | https://gsrs.ncats.nih.gov/ginas/app/beta/substances/0VFV53RMC2 | |
Description | The FDA Global Substance Registration System (GSRS) enables the efficient and accurate exchange of information on what substances are in regulated products. Instead of relying on names, which vary across regulatory domains, countries, and regions, the GSRS knowledge base makes it possible for substances to be defined by standardized, scientific descriptions. | |
Explanation | Unless otherwise noted, the contents of the FDA website (www.fda.gov), both text and graphics, are not copyrighted. They are in the public domain and may be republished, reprinted and otherwise used freely by anyone without the need to obtain permission from FDA. Credit to the U.S. Food and Drug Administration as the source is appreciated but not required. | |
Retrosynthesis Analysis
AI-Powered Synthesis Planning: Our tool employs the Template_relevance Pistachio, Template_relevance Bkms_metabolic, Template_relevance Pistachio_ringbreaker, Template_relevance Reaxys, Template_relevance Reaxys_biocatalysis model, leveraging a vast database of chemical reactions to predict feasible synthetic routes.
One-Step Synthesis Focus: Specifically designed for one-step synthesis, it provides concise and direct routes for your target compounds, streamlining the synthesis process.
Accurate Predictions: Utilizing the extensive PISTACHIO, BKMS_METABOLIC, PISTACHIO_RINGBREAKER, REAXYS, REAXYS_BIOCATALYSIS database, our tool offers high-accuracy predictions, reflecting the latest in chemical research and data.
Strategy Settings
Precursor scoring | Relevance Heuristic |
---|---|
Min. plausibility | 0.01 |
Model | Template_relevance |
Template Set | Pistachio/Bkms_metabolic/Pistachio_ringbreaker/Reaxys/Reaxys_biocatalysis |
Top-N result to add to graph | 6 |
Feasible Synthetic Routes
Disclaimer and Information on In-Vitro Research Products
Please be aware that all articles and product information presented on BenchChem are intended solely for informational purposes. The products available for purchase on BenchChem are specifically designed for in-vitro studies, which are conducted outside of living organisms. In-vitro studies, derived from the Latin term "in glass," involve experiments performed in controlled laboratory settings using cells or tissues. It is important to note that these products are not categorized as medicines or drugs, and they have not received approval from the FDA for the prevention, treatment, or cure of any medical condition, ailment, or disease. We must emphasize that any form of bodily introduction of these products into humans or animals is strictly prohibited by law. It is essential to adhere to these guidelines to ensure compliance with legal and ethical standards in research and experimentation.