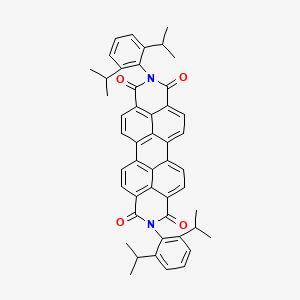
N,N'-双(2,6-二异丙苯基)-3,4,9,10-苝四甲酸二酰亚胺
描述
“N,N’-Bis(2,6-diisopropylphenyl)-3,4,9,10-perylenetetracarboxylic Diimide” is a perylene-based organic photocatalyst . It has a variety of demonstrated uses, including the reduction of aryl halides and the iodoperfluoroalkylation of alkenes .
Molecular Structure Analysis
The π-conjugated perylene skeleton of “N,N’-Bis(2,6-diisopropylphenyl)-3,4,9,10-perylenetetracarboxylic Diimide” is nearly planar . The molecular formula is C48H42N2O4 .Physical And Chemical Properties Analysis
“N,N’-Bis(2,6-diisopropylphenyl)-3,4,9,10-perylenetetracarboxylic Diimide” is a powder with a melting point greater than 300°C . It has an empirical formula of C48H42N2O4 and a molecular weight of 710.86 .科学研究应用
有机场效应晶体管 (OFET) 制造
一系列烷基或烷基苯基取代的萘四甲酸和苝四甲酸双酰亚胺已被合成和表征。这些化合物,包括 N,N'-双(2,6-二异丙苯基)-3,4,9,10-苝四甲酸二酰亚胺,表现出改善的溶液可加工性。这一特性使其适用于通过旋涂和印刷技术制造全有机柔性 n 沟道场效应晶体管 (OFET),而无需真空沉积。合成的有机半导体可以实现 4 × 10^−2 cm^2/(V·s) 的电荷载流子迁移率和 4.5 × 10^5 的开/关比,展示了它们在 OFET 应用中的潜力 (Gawryś 等,2009)。
晶体结构和热电性能
N,N'-双(2,6-二异丙苯基)-3,4,9,10-苝四甲酸二酰亚胺 (PDI) 及其衍生物的晶体结构已被报道,揭示了它们的 π 共轭苝骨架结构。由于取代,这些结构表现出不同的平面度和扭曲,这会影响它们的固态堆积和热电性能。这种结构理解为针对特定应用定制 PDI 衍生物的电子和热电性能提供了基础 (Aksakal 等,2018)。
电活性聚合物网络
低聚噻吩基官能化的苝双酰亚胺,包括 N,N'-双(2,6-二异丙苯基)-3,4,9,10-苝四甲酸二酰亚胺变体,已被合成并研究其电活性。这些化合物显示出特征性的紫外/可见吸收特性,并在激发后促进荧光共振能量转移 (FRET) 到苝双酰亚胺核心中。它们还参与氧化偶联,从而形成电活性网络,展示了它们在制造 p 型导电材料中的潜力 (You 等,2007)。
光伏应用
N,N'-双(2,6-二异丙苯基)-3,4,9,10-苝四甲酸二酰亚胺的新衍生物已被合成用于光伏应用。这些分子旨在减少分子聚集并提高溶解度,已用作本体异质结太阳能电池中的受体组件,展示了它们在开发高效光伏器件中的作用 (Kozma 等,2013)。
安全和危害
作用机制
Target of Action
It’s known that perylene orange is a perylene diimide (pdi)-based molecule . PDI-based molecules are known for their excellent thermal, chemical, and photochemical stability, unique UV-vis absorption, and fluorescent emission properties .
Mode of Action
Perylene Orange interacts with its targets through a process known as Förster energy transfer . This process involves the non-radiative transfer of energy from a donor molecule (in this case, Perylene Orange) to an acceptor molecule . The energy transfer results in a change in the fluorescence of the Perylene Orange, with a strong intensity drop observed when the molecules are brought onto monolayer transition metal dichalcogenides (TMDCs) via thermal vapor deposition .
Biochemical Pathways
The energy transfer process it facilitates can influence various biochemical processes, particularly those involving fluorescence or colorimetric changes .
Pharmacokinetics
Its unique uv-vis absorption and fluorescent emission properties suggest potential bioavailability .
Result of Action
The primary result of Perylene Orange’s action is a change in fluorescence. This change is due to the energy transfer from Perylene Orange to other molecules, resulting in a strong intensity drop of the Perylene Orange fluorescence . This property makes Perylene Orange useful in applications such as optoelectronic devices .
Action Environment
Environmental factors can significantly influence the action, efficacy, and stability of Perylene Orange. For instance, the fluorescence of Perylene Orange can be quenched or enhanced depending on the presence of other molecules in its environment . Additionally, the thermal vapor deposition process used to bring Perylene Orange molecules onto monolayer TMDCs can also influence its action .
属性
IUPAC Name |
7,18-bis[2,6-di(propan-2-yl)phenyl]-7,18-diazaheptacyclo[14.6.2.22,5.03,12.04,9.013,23.020,24]hexacosa-1(23),2,4,9,11,13,15,20(24),21,25-decaene-6,8,17,19-tetrone | |
---|---|---|
Source | PubChem | |
URL | https://pubchem.ncbi.nlm.nih.gov | |
Description | Data deposited in or computed by PubChem | |
InChI |
InChI=1S/C48H42N2O4/c1-23(2)27-11-9-12-28(24(3)4)43(27)49-45(51)35-19-15-31-33-17-21-37-42-38(22-18-34(40(33)42)32-16-20-36(46(49)52)41(35)39(31)32)48(54)50(47(37)53)44-29(25(5)6)13-10-14-30(44)26(7)8/h9-26H,1-8H3 | |
Source | PubChem | |
URL | https://pubchem.ncbi.nlm.nih.gov | |
Description | Data deposited in or computed by PubChem | |
InChI Key |
NAZODJSYHDYJGP-UHFFFAOYSA-N | |
Source | PubChem | |
URL | https://pubchem.ncbi.nlm.nih.gov | |
Description | Data deposited in or computed by PubChem | |
Canonical SMILES |
CC(C)C1=C(C(=CC=C1)C(C)C)N2C(=O)C3=C4C(=CC=C5C4=C(C=C3)C6=C7C5=CC=C8C7=C(C=C6)C(=O)N(C8=O)C9=C(C=CC=C9C(C)C)C(C)C)C2=O | |
Source | PubChem | |
URL | https://pubchem.ncbi.nlm.nih.gov | |
Description | Data deposited in or computed by PubChem | |
Molecular Formula |
C48H42N2O4 | |
Source | PubChem | |
URL | https://pubchem.ncbi.nlm.nih.gov | |
Description | Data deposited in or computed by PubChem | |
Molecular Weight |
710.9 g/mol | |
Source | PubChem | |
URL | https://pubchem.ncbi.nlm.nih.gov | |
Description | Data deposited in or computed by PubChem | |
Product Name |
N,N'-Bis(2,6-diisopropylphenyl)-3,4,9,10-perylenetetracarboxylic Diimide |
Retrosynthesis Analysis
AI-Powered Synthesis Planning: Our tool employs the Template_relevance Pistachio, Template_relevance Bkms_metabolic, Template_relevance Pistachio_ringbreaker, Template_relevance Reaxys, Template_relevance Reaxys_biocatalysis model, leveraging a vast database of chemical reactions to predict feasible synthetic routes.
One-Step Synthesis Focus: Specifically designed for one-step synthesis, it provides concise and direct routes for your target compounds, streamlining the synthesis process.
Accurate Predictions: Utilizing the extensive PISTACHIO, BKMS_METABOLIC, PISTACHIO_RINGBREAKER, REAXYS, REAXYS_BIOCATALYSIS database, our tool offers high-accuracy predictions, reflecting the latest in chemical research and data.
Strategy Settings
Precursor scoring | Relevance Heuristic |
---|---|
Min. plausibility | 0.01 |
Model | Template_relevance |
Template Set | Pistachio/Bkms_metabolic/Pistachio_ringbreaker/Reaxys/Reaxys_biocatalysis |
Top-N result to add to graph | 6 |
Feasible Synthetic Routes
Q & A
Q1: What is the molecular formula and weight of Perylene Orange?
A1: Perylene Orange, also known as N,N'-Bis(2,6-diisopropylphenyl)-3,4,9,10-perylenetetracarboxylic Diimide, has the molecular formula C44H38N2O4 and a molecular weight of 658.77 g/mol.
Q2: What spectroscopic data is available for Perylene Orange?
A2: Perylene Orange exhibits characteristic absorption and emission spectra. It typically shows absorption maxima around 468 nm and 499 nm. [] The fluorescence emission maximum is typically observed around 578 nm. [] Detailed spectral analysis, including UV-Vis and fluorescence spectra, can be found in various research papers. [, , , , , , ]
Q3: Which materials are commonly used as hosts for Perylene Orange in solid-state applications?
A3: Perylene Orange has been successfully incorporated into various host matrices for solid-state applications, including:
- Poly(methyl methacrylate) (PMMA) [, , , , , , ]
- Sol-gel glass [, , , ]
- Polycom glass (sol-gel glass-PMMA composite) [, ]
- Polystyrene (PS) [, , ]
- Acrylic polymer [, ]
- AlPO4 mesoporous glass []
- Organically modified silicate (Ormosil) glass []
- Fluoro-modified thermoplastic resist mr-I7030R []
Q4: How does the choice of host matrix affect the stability and performance of Perylene Orange?
A4: The photostability and laser performance of Perylene Orange are significantly influenced by the surrounding chemical environment provided by the host matrix. For instance, Perylene Orange exhibits enhanced photostability in oxygen-free environments. [] Additionally, its performance is notably better in partially organic hosts compared to purely inorganic ones. [] The specific interactions between Perylene Orange and the host matrix, such as oxygen permeability and thermal properties, play a crucial role in determining its overall stability and effectiveness in various applications. []
Q5: How does oxygen affect the photostability of Perylene Orange?
A5: Dissolved oxygen has been found to accelerate the photodegradation of Perylene Orange in solution. [] This degradation process is significantly reduced in oxygen-free environments, leading to enhanced photostability and longer operational lifetimes for Perylene Orange-based devices. [, ]
Q6: What factors influence the formation of aggregates in Perylene Orange solutions?
A6: The formation of Perylene Orange aggregates, such as J-dimers, is influenced by factors like dipping time and concentration. [] Longer dipping times and higher concentrations can lead to increased aggregation. []
Q7: What is the impact of Perylene Orange concentration on its fluorescence properties in AlPO4 mesoporous glass?
A7: Increasing the concentration of Perylene Orange in AlPO4 mesoporous glass can lead to a slight red shift in both excitation and emission spectra. [] This shift is attributed to the formation of aggregates, such as J-dimers, at higher concentrations. []
Q8: What is the mechanism of energy transfer in Perylene Orange systems?
A8: Perylene Orange exhibits efficient energy transfer primarily through the Förster resonance energy transfer (FRET) mechanism. [, , , , ] This non-radiative process involves the transfer of energy from an excited donor molecule (Perylene Orange) to a nearby acceptor molecule through dipole-dipole interactions. [, , ]
Q9: How efficient is the energy transfer process in Perylene Orange systems?
A9: Energy transfer in Perylene Orange systems can be remarkably efficient, with transfer times as fast as 600 fs reported for heterotransfer to Perylene Red. [] This ultrafast energy transfer contributes to the high exciton mobility observed in highly doped Perylene Orange matrixes. []
Q10: What is the role of exciton migration in Perylene Orange systems?
A10: Exciton migration, facilitated by efficient energy transfer between Perylene Orange molecules, plays a crucial role in transporting and collecting energy within these systems. [] This efficient energy transport is particularly advantageous for applications in organic electronic devices and molecular systems. []
Q11: What are the primary applications of Perylene Orange?
A11: Perylene Orange is primarily investigated and utilized for its excellent luminescent properties, particularly in the development of:
- Solid-State Dye Lasers (SSDLs): Perylene Orange is a promising laser dye due to its high fluorescence quantum yield, broad absorption and emission spectra, and good photostability, especially when incorporated into suitable solid host matrices. [, , , , , , , , , , ]
- Sensors: Perylene Orange-doped materials have shown potential for use in refractive index sensors, where changes in the emission wavelength can be correlated to changes in the surrounding refractive index. []
- Fluorescence Microscopy: Perylene Orange has been used as a fluorescent probe in single-molecule fluorescence microscopy studies, providing insights into its photophysical properties and interactions with its surrounding environment. [, , ]
Q12: How does the laser performance of Perylene Orange compare to other dyes?
A12: Perylene Orange has demonstrated comparable and even superior laser performance compared to other commonly used laser dyes like Rhodamine 590 and Pyrromethene 567. [, ] Its high slope efficiency, low lasing threshold, and excellent photostability in certain matrices make it a strong candidate for SSDL applications. [, , ]
Q13: How does the photostability of Perylene Orange in solid matrices compare to that in solution?
A13: Perylene Orange generally exhibits enhanced photostability when trapped in solid matrices compared to when it is dissolved in solution. [] This enhanced stability is attributed to the restricted movement and reduced interaction with oxygen within the solid matrix, mitigating photodegradation pathways. []
Q14: Have computational methods been used to study Perylene Orange?
A14: Yes, computational chemistry techniques like Density Functional Theory (DFT) and Time-Dependent DFT (TD-DFT) have been employed to investigate the structural and electronic properties of Perylene Orange and its complexes. [] These methods help predict UV-Vis spectra, optimize molecular geometries, and explore intermolecular interactions, contributing to a deeper understanding of its photophysical behavior. [, ]
Q15: What insights have molecular dynamics (MD) simulations provided into the aggregation behavior of Perylene Orange?
A15: MD simulations have revealed that Perylene Orange, despite its bulky imide groups, can exhibit complex aggregation behavior beyond simple π-stacking interactions. [] Simulations indicate the presence of an energetically favorable "edge-to-edge" aggregate structure, challenging previous assumptions about dominant aggregation mechanisms in PDI systems. []
体外研究产品的免责声明和信息
请注意,BenchChem 上展示的所有文章和产品信息仅供信息参考。 BenchChem 上可购买的产品专为体外研究设计,这些研究在生物体外进行。体外研究,源自拉丁语 "in glass",涉及在受控实验室环境中使用细胞或组织进行的实验。重要的是要注意,这些产品没有被归类为药物或药品,他们没有得到 FDA 的批准,用于预防、治疗或治愈任何医疗状况、疾病或疾病。我们必须强调,将这些产品以任何形式引入人类或动物的身体都是法律严格禁止的。遵守这些指南对确保研究和实验的法律和道德标准的符合性至关重要。