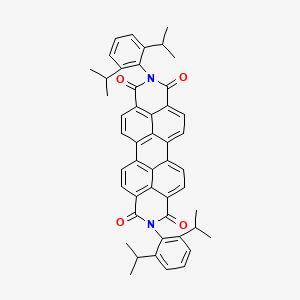
N,N'-Bis(2,6-diisopropylphenyl)-3,4,9,10-perylenetetracarboxylic Diimide
Overview
Description
“N,N’-Bis(2,6-diisopropylphenyl)-3,4,9,10-perylenetetracarboxylic Diimide” is a perylene-based organic photocatalyst . It has a variety of demonstrated uses, including the reduction of aryl halides and the iodoperfluoroalkylation of alkenes .
Molecular Structure Analysis
The π-conjugated perylene skeleton of “N,N’-Bis(2,6-diisopropylphenyl)-3,4,9,10-perylenetetracarboxylic Diimide” is nearly planar . The molecular formula is C48H42N2O4 .Physical And Chemical Properties Analysis
“N,N’-Bis(2,6-diisopropylphenyl)-3,4,9,10-perylenetetracarboxylic Diimide” is a powder with a melting point greater than 300°C . It has an empirical formula of C48H42N2O4 and a molecular weight of 710.86 .Scientific Research Applications
Organic Field Effect Transistors (OFETs) Fabrication
A series of alkyl- or alkylphenyl-substituted naphthalenetetracarboxylic and perylenetetracarboxylic bisimides have been synthesized and characterized. These compounds, including N,N'-Bis(2,6-diisopropylphenyl)-3,4,9,10-perylenetetracarboxylic diimide, exhibit improved solution processibility. This characteristic makes them suitable for the fabrication of all-organic, flexible n-channel field effect transistors (OFETs) through spin coating and printing techniques, without the necessity of vacuum deposition. The synthesized organic semiconductors can achieve charge carriers mobility of 4 × 10^−2 cm^2/(V·s) and an ON/OFF ratio equal to 4.5 × 10^5, demonstrating their potential in OFETs applications (Gawryś et al., 2009).
Crystal Structure and Thermoelectric Properties
The crystal structures of N,N'-bis(2,6-diisopropylphenyl)-3,4,9,10-perylenetetracarboxylic diimide (PDI) and its derivatives have been reported, revealing their π-conjugated perylene skeleton structures. Such structures exhibit different planarity and twisting due to substitution, which impacts their solid-state packing and thermoelectric properties. This structural understanding provides a basis for tailoring the electronic and thermoelectric properties of PDI derivatives for specific applications (Aksakal et al., 2018).
Electroactive Polymeric Networks
Oligothienyl-functionalized perylene bisimides, including N,N'-bis(2,6-diisopropylphenyl)-3,4,9,10-perylenetetracarboxylic diimide variants, have been synthesized and studied for their electroactive properties. These compounds display characteristic UV/vis absorption properties and facilitate fluorescence resonance energy transfer (FRET) to the perylene bisimide core upon excitation. They also participate in oxidative coupling leading to electroactive networks, demonstrating their potential in creating p-type conductive materials (You et al., 2007).
Photovoltaic Applications
New derivatives of N,N'-Bis(2,6-diisopropylphenyl)-3,4,9,10-perylenetetracarboxylic diimide have been synthesized for photovoltaic applications. These molecules, designed to reduce molecular aggregation and enhance solubility, have been utilized as acceptor components in bulk heterojunction solar cells, showcasing their role in the development of efficient photovoltaic devices (Kozma et al., 2013).
Safety and Hazards
Mechanism of Action
Target of Action
It’s known that perylene orange is a perylene diimide (pdi)-based molecule . PDI-based molecules are known for their excellent thermal, chemical, and photochemical stability, unique UV-vis absorption, and fluorescent emission properties .
Mode of Action
Perylene Orange interacts with its targets through a process known as Förster energy transfer . This process involves the non-radiative transfer of energy from a donor molecule (in this case, Perylene Orange) to an acceptor molecule . The energy transfer results in a change in the fluorescence of the Perylene Orange, with a strong intensity drop observed when the molecules are brought onto monolayer transition metal dichalcogenides (TMDCs) via thermal vapor deposition .
Biochemical Pathways
The energy transfer process it facilitates can influence various biochemical processes, particularly those involving fluorescence or colorimetric changes .
Pharmacokinetics
Its unique uv-vis absorption and fluorescent emission properties suggest potential bioavailability .
Result of Action
The primary result of Perylene Orange’s action is a change in fluorescence. This change is due to the energy transfer from Perylene Orange to other molecules, resulting in a strong intensity drop of the Perylene Orange fluorescence . This property makes Perylene Orange useful in applications such as optoelectronic devices .
Action Environment
Environmental factors can significantly influence the action, efficacy, and stability of Perylene Orange. For instance, the fluorescence of Perylene Orange can be quenched or enhanced depending on the presence of other molecules in its environment . Additionally, the thermal vapor deposition process used to bring Perylene Orange molecules onto monolayer TMDCs can also influence its action .
properties
IUPAC Name |
7,18-bis[2,6-di(propan-2-yl)phenyl]-7,18-diazaheptacyclo[14.6.2.22,5.03,12.04,9.013,23.020,24]hexacosa-1(23),2,4,9,11,13,15,20(24),21,25-decaene-6,8,17,19-tetrone | |
---|---|---|
Source | PubChem | |
URL | https://pubchem.ncbi.nlm.nih.gov | |
Description | Data deposited in or computed by PubChem | |
InChI |
InChI=1S/C48H42N2O4/c1-23(2)27-11-9-12-28(24(3)4)43(27)49-45(51)35-19-15-31-33-17-21-37-42-38(22-18-34(40(33)42)32-16-20-36(46(49)52)41(35)39(31)32)48(54)50(47(37)53)44-29(25(5)6)13-10-14-30(44)26(7)8/h9-26H,1-8H3 | |
Source | PubChem | |
URL | https://pubchem.ncbi.nlm.nih.gov | |
Description | Data deposited in or computed by PubChem | |
InChI Key |
NAZODJSYHDYJGP-UHFFFAOYSA-N | |
Source | PubChem | |
URL | https://pubchem.ncbi.nlm.nih.gov | |
Description | Data deposited in or computed by PubChem | |
Canonical SMILES |
CC(C)C1=C(C(=CC=C1)C(C)C)N2C(=O)C3=C4C(=CC=C5C4=C(C=C3)C6=C7C5=CC=C8C7=C(C=C6)C(=O)N(C8=O)C9=C(C=CC=C9C(C)C)C(C)C)C2=O | |
Source | PubChem | |
URL | https://pubchem.ncbi.nlm.nih.gov | |
Description | Data deposited in or computed by PubChem | |
Molecular Formula |
C48H42N2O4 | |
Source | PubChem | |
URL | https://pubchem.ncbi.nlm.nih.gov | |
Description | Data deposited in or computed by PubChem | |
Molecular Weight |
710.9 g/mol | |
Source | PubChem | |
URL | https://pubchem.ncbi.nlm.nih.gov | |
Description | Data deposited in or computed by PubChem | |
Product Name |
N,N'-Bis(2,6-diisopropylphenyl)-3,4,9,10-perylenetetracarboxylic Diimide |
Retrosynthesis Analysis
AI-Powered Synthesis Planning: Our tool employs the Template_relevance Pistachio, Template_relevance Bkms_metabolic, Template_relevance Pistachio_ringbreaker, Template_relevance Reaxys, Template_relevance Reaxys_biocatalysis model, leveraging a vast database of chemical reactions to predict feasible synthetic routes.
One-Step Synthesis Focus: Specifically designed for one-step synthesis, it provides concise and direct routes for your target compounds, streamlining the synthesis process.
Accurate Predictions: Utilizing the extensive PISTACHIO, BKMS_METABOLIC, PISTACHIO_RINGBREAKER, REAXYS, REAXYS_BIOCATALYSIS database, our tool offers high-accuracy predictions, reflecting the latest in chemical research and data.
Strategy Settings
Precursor scoring | Relevance Heuristic |
---|---|
Min. plausibility | 0.01 |
Model | Template_relevance |
Template Set | Pistachio/Bkms_metabolic/Pistachio_ringbreaker/Reaxys/Reaxys_biocatalysis |
Top-N result to add to graph | 6 |
Feasible Synthetic Routes
Q & A
Q1: What is the molecular formula and weight of Perylene Orange?
A1: Perylene Orange, also known as N,N'-Bis(2,6-diisopropylphenyl)-3,4,9,10-perylenetetracarboxylic Diimide, has the molecular formula C44H38N2O4 and a molecular weight of 658.77 g/mol.
Q2: What spectroscopic data is available for Perylene Orange?
A2: Perylene Orange exhibits characteristic absorption and emission spectra. It typically shows absorption maxima around 468 nm and 499 nm. [] The fluorescence emission maximum is typically observed around 578 nm. [] Detailed spectral analysis, including UV-Vis and fluorescence spectra, can be found in various research papers. [, , , , , , ]
Q3: Which materials are commonly used as hosts for Perylene Orange in solid-state applications?
A3: Perylene Orange has been successfully incorporated into various host matrices for solid-state applications, including:
- Poly(methyl methacrylate) (PMMA) [, , , , , , ]
- Sol-gel glass [, , , ]
- Polycom glass (sol-gel glass-PMMA composite) [, ]
- Polystyrene (PS) [, , ]
- Acrylic polymer [, ]
- AlPO4 mesoporous glass []
- Organically modified silicate (Ormosil) glass []
- Fluoro-modified thermoplastic resist mr-I7030R []
Q4: How does the choice of host matrix affect the stability and performance of Perylene Orange?
A4: The photostability and laser performance of Perylene Orange are significantly influenced by the surrounding chemical environment provided by the host matrix. For instance, Perylene Orange exhibits enhanced photostability in oxygen-free environments. [] Additionally, its performance is notably better in partially organic hosts compared to purely inorganic ones. [] The specific interactions between Perylene Orange and the host matrix, such as oxygen permeability and thermal properties, play a crucial role in determining its overall stability and effectiveness in various applications. []
Q5: How does oxygen affect the photostability of Perylene Orange?
A5: Dissolved oxygen has been found to accelerate the photodegradation of Perylene Orange in solution. [] This degradation process is significantly reduced in oxygen-free environments, leading to enhanced photostability and longer operational lifetimes for Perylene Orange-based devices. [, ]
Q6: What factors influence the formation of aggregates in Perylene Orange solutions?
A6: The formation of Perylene Orange aggregates, such as J-dimers, is influenced by factors like dipping time and concentration. [] Longer dipping times and higher concentrations can lead to increased aggregation. []
Q7: What is the impact of Perylene Orange concentration on its fluorescence properties in AlPO4 mesoporous glass?
A7: Increasing the concentration of Perylene Orange in AlPO4 mesoporous glass can lead to a slight red shift in both excitation and emission spectra. [] This shift is attributed to the formation of aggregates, such as J-dimers, at higher concentrations. []
Q8: What is the mechanism of energy transfer in Perylene Orange systems?
A8: Perylene Orange exhibits efficient energy transfer primarily through the Förster resonance energy transfer (FRET) mechanism. [, , , , ] This non-radiative process involves the transfer of energy from an excited donor molecule (Perylene Orange) to a nearby acceptor molecule through dipole-dipole interactions. [, , ]
Q9: How efficient is the energy transfer process in Perylene Orange systems?
A9: Energy transfer in Perylene Orange systems can be remarkably efficient, with transfer times as fast as 600 fs reported for heterotransfer to Perylene Red. [] This ultrafast energy transfer contributes to the high exciton mobility observed in highly doped Perylene Orange matrixes. []
Q10: What is the role of exciton migration in Perylene Orange systems?
A10: Exciton migration, facilitated by efficient energy transfer between Perylene Orange molecules, plays a crucial role in transporting and collecting energy within these systems. [] This efficient energy transport is particularly advantageous for applications in organic electronic devices and molecular systems. []
Q11: What are the primary applications of Perylene Orange?
A11: Perylene Orange is primarily investigated and utilized for its excellent luminescent properties, particularly in the development of:
- Solid-State Dye Lasers (SSDLs): Perylene Orange is a promising laser dye due to its high fluorescence quantum yield, broad absorption and emission spectra, and good photostability, especially when incorporated into suitable solid host matrices. [, , , , , , , , , , ]
- Sensors: Perylene Orange-doped materials have shown potential for use in refractive index sensors, where changes in the emission wavelength can be correlated to changes in the surrounding refractive index. []
- Fluorescence Microscopy: Perylene Orange has been used as a fluorescent probe in single-molecule fluorescence microscopy studies, providing insights into its photophysical properties and interactions with its surrounding environment. [, , ]
Q12: How does the laser performance of Perylene Orange compare to other dyes?
A12: Perylene Orange has demonstrated comparable and even superior laser performance compared to other commonly used laser dyes like Rhodamine 590 and Pyrromethene 567. [, ] Its high slope efficiency, low lasing threshold, and excellent photostability in certain matrices make it a strong candidate for SSDL applications. [, , ]
Q13: How does the photostability of Perylene Orange in solid matrices compare to that in solution?
A13: Perylene Orange generally exhibits enhanced photostability when trapped in solid matrices compared to when it is dissolved in solution. [] This enhanced stability is attributed to the restricted movement and reduced interaction with oxygen within the solid matrix, mitigating photodegradation pathways. []
Q14: Have computational methods been used to study Perylene Orange?
A14: Yes, computational chemistry techniques like Density Functional Theory (DFT) and Time-Dependent DFT (TD-DFT) have been employed to investigate the structural and electronic properties of Perylene Orange and its complexes. [] These methods help predict UV-Vis spectra, optimize molecular geometries, and explore intermolecular interactions, contributing to a deeper understanding of its photophysical behavior. [, ]
Q15: What insights have molecular dynamics (MD) simulations provided into the aggregation behavior of Perylene Orange?
A15: MD simulations have revealed that Perylene Orange, despite its bulky imide groups, can exhibit complex aggregation behavior beyond simple π-stacking interactions. [] Simulations indicate the presence of an energetically favorable "edge-to-edge" aggregate structure, challenging previous assumptions about dominant aggregation mechanisms in PDI systems. []
Disclaimer and Information on In-Vitro Research Products
Please be aware that all articles and product information presented on BenchChem are intended solely for informational purposes. The products available for purchase on BenchChem are specifically designed for in-vitro studies, which are conducted outside of living organisms. In-vitro studies, derived from the Latin term "in glass," involve experiments performed in controlled laboratory settings using cells or tissues. It is important to note that these products are not categorized as medicines or drugs, and they have not received approval from the FDA for the prevention, treatment, or cure of any medical condition, ailment, or disease. We must emphasize that any form of bodily introduction of these products into humans or animals is strictly prohibited by law. It is essential to adhere to these guidelines to ensure compliance with legal and ethical standards in research and experimentation.