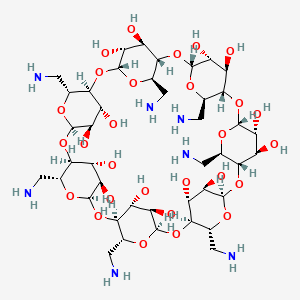
Per-6-amino-beta-cyclodextrin
説明
Per-6-amino-beta-cyclodextrin is a derivative of beta-cyclodextrin, a naturally occurring oligosaccharide consisting of six, seven, or eight glucose units . The amino group is attached to the primary face of the beta-cyclodextrin torus . This compound has been used in various applications, including as a catalyst in chemical reactions .
Synthesis Analysis
The synthesis of this compound involves coupling amino acids to the primary face of the beta-cyclodextrin torus. This has been achieved using the backbone carboxylic acid functionality of phenylalanine and the side chain thiol group of cysteine . In the case of the heptacysteinyl derivatives, polyzwitterionic compounds were obtained and shown to be highly water soluble .
Molecular Structure Analysis
This compound has been studied using density functional theory (DFT) method. The interaction between this compound and benzaldehyde or o-aminoacetophenone plays an important role in this reaction .
Chemical Reactions Analysis
This compound has been used as a catalyst in the synthesis of 2-aryl-2,3-dihydro-4-quinolones . The interaction between this compound and benzaldehyde or o-aminoacetophenone plays an important role in this reaction .
Physical and Chemical Properties Analysis
This compound is a derivative of beta-cyclodextrin, a naturally occurring oligosaccharide consisting of six, seven, or eight glucose units . The amino group is attached to the primary face of the beta-cyclodextrin torus . This compound has been used in various applications, including as a catalyst in chemical reactions .
科学的研究の応用
1. Catalysis in Chemical Synthesis
Per-6-ABCD serves as an efficient supramolecular ligand and host in the Cu(I)-catalyzed N-arylation of imidazole with aryl bromides. This process demonstrates good tolerance of other functionalities and proceeds with excellent yield, thus showing its potential in facilitating chemical synthesis under mild conditions (Suresh & Pitchumani, 2008).
2. Inclusion Properties in Peptides
β-Cyclodextrins, including Per-6-ABCD, are known for their inclusion properties with peptides. They impact protein thermal and proteolytic stability, refolding yields, solubility, and taste masking. The inclusion properties of β-Cyclodextrin with aromatic amino acids in peptides have been investigated, revealing significant effects on peptide binding constants (Caso et al., 2015).
3. Green Chemistry Applications
Per-6-ABCD is used as a supramolecular host and efficient solid base catalyst in solvent-free syntheses of various compounds, such as dihydropyrano[2,3-c]pyrazole derivatives. This method is characterized by its atom-economical, mild, and green approach, aligning with sustainability goals in chemistry (Kanagaraj & Pitchumani, 2010).
4. Supramolecular Chemistry
Per-6-ABCD plays a role in supramolecular chemistry, particularly in the formation of complex structures like micelles, vesicles, and nanoparticles. These structures are significant in various fields, including pharmaceuticals and material science (Varan et al., 2017).
作用機序
Target of Action
Per-6-amino-beta-cyclodextrin (PA-β-CD) primarily targets aryl halides and imidazole . It acts as a supramolecular ligand for CuI and a host for aryl bromides .
Mode of Action
PA-β-CD interacts with its targets to catalyze specific reactions. For instance, it catalyzes the N-arylation of imidazole with aryl bromides under mild conditions . It also promotes the one-pot synthesis of enantiomerically-enriched 2-aryl-2,3-dihydroquinolin-4(1H)-ones .
Biochemical Pathways
PA-β-CD affects the cyanation of aryl halides . It uses less toxic K4 [Fe (CN)6] as the reagent and amino-β-cyclodextrins as supramolecular ligands for CuI . This leads to the production of aryl and heteroaryl nitriles .
Result of Action
The action of PA-β-CD results in the synthesis of enantiomerically-enriched 2-aryl-2,3-dihydroquinolin-4(1H)-ones with high yield (up to 99%) and enantiomeric excess (up to 99%) . It also leads to the efficient cyanation of aryl halides .
Action Environment
The action of PA-β-CD is influenced by environmental factors. For instance, it can catalyze reactions under mild conditions . , suggesting that they can function effectively in aqueous environments.
将来の方向性
生化学分析
Biochemical Properties
Per-6-amino-beta-cyclodextrin plays a crucial role in biochemical reactions, primarily as a supramolecular ligand and host. It interacts with various enzymes, proteins, and other biomolecules, facilitating catalytic processes and enhancing reaction efficiency. For instance, this compound acts as a supramolecular ligand for copper(I) ions, catalyzing the N-arylation of imidazole with aryl bromides under mild conditions . This interaction demonstrates the compound’s ability to stabilize transition states and improve reaction yields. Additionally, this compound forms inclusion complexes with hydrophobic molecules, thereby enhancing their solubility and bioavailability .
Cellular Effects
This compound influences various cellular processes, including cell signaling pathways, gene expression, and cellular metabolism. It has been observed to interact with cell membranes, altering their cholesterol content and affecting membrane fluidity . This interaction can disrupt the barrier function of cell layers, leading to changes in cellular permeability and transport. Furthermore, this compound has been shown to modulate gene expression by forming complexes with transcription factors and other regulatory proteins, thereby influencing cellular responses to external stimuli .
Molecular Mechanism
The molecular mechanism of this compound involves its ability to form stable inclusion complexes with various biomolecules. This compound acts as a catalyst in several organic reactions by providing a suitable microenvironment for the reactants. For example, this compound catalyzes the synthesis of 2-aryl-2,3-dihydro-4-quinolones by interacting with benzaldehyde and o-aminoacetophenone . The formation of hydrogen bonds and donor-acceptor interactions within the cyclodextrin cavity enhances the reaction’s selectivity and yield. Additionally, this compound can inhibit or activate enzymes by binding to their active sites, thereby modulating their activity .
Temporal Effects in Laboratory Settings
In laboratory settings, the effects of this compound can change over time due to its stability and degradation properties. Studies have shown that this compound remains stable under various conditions, maintaining its catalytic activity over extended periods . Prolonged exposure to certain environmental factors, such as high temperatures or extreme pH levels, can lead to its degradation and reduced efficacy. Long-term studies have also indicated that this compound can have lasting effects on cellular function, including sustained changes in gene expression and metabolic activity .
Dosage Effects in Animal Models
The effects of this compound vary with different dosages in animal models. At lower doses, the compound has been shown to enhance the bioavailability and efficacy of co-administered drugs by forming inclusion complexes . At higher doses, this compound can exhibit toxic or adverse effects, such as disrupting cellular membranes and causing cytotoxicity . Threshold effects have been observed, where the compound’s beneficial properties are maximized at optimal dosages, while excessive amounts lead to detrimental outcomes.
Metabolic Pathways
This compound is involved in various metabolic pathways, interacting with enzymes and cofactors to modulate metabolic flux and metabolite levels. The compound has been shown to participate in the metabolism of hydrophobic molecules by forming inclusion complexes that facilitate their transport and degradation . Additionally, this compound can influence the activity of metabolic enzymes by binding to their active sites, thereby altering their catalytic efficiency and substrate specificity .
Transport and Distribution
Within cells and tissues, this compound is transported and distributed through interactions with specific transporters and binding proteins. The compound’s hydrophilic exterior and hydrophobic cavity enable it to traverse cellular membranes and accumulate in target tissues . This compound can also interact with membrane-bound receptors and transporters, facilitating its uptake and distribution within the cell . These interactions play a crucial role in determining the compound’s localization and bioavailability.
Subcellular Localization
This compound exhibits specific subcellular localization, which influences its activity and function. The compound is often directed to particular cellular compartments or organelles through targeting signals or post-translational modifications . For instance, this compound can localize to the endoplasmic reticulum or mitochondria, where it interacts with resident proteins and modulates their function . This targeted localization enhances the compound’s efficacy in catalyzing biochemical reactions and regulating cellular processes.
特性
IUPAC Name |
(1S,3R,5R,6S,8R,10R,11S,13R,15R,16S,18R,20R,21S,23R,25R,26S,28R,30R,31S,33R,35R,36R,37R,38R,39R,40R,41R,42R,43R,44R,45R,46R,47R,48R,49R)-5,10,15,20,25,30,35-heptakis(aminomethyl)-2,4,7,9,12,14,17,19,22,24,27,29,32,34-tetradecaoxaoctacyclo[31.2.2.23,6.28,11.213,16.218,21.223,26.228,31]nonatetracontane-36,37,38,39,40,41,42,43,44,45,46,47,48,49-tetradecol | |
---|---|---|
Details | Computed by Lexichem TK 2.7.0 (PubChem release 2021.05.07) | |
Source | PubChem | |
URL | https://pubchem.ncbi.nlm.nih.gov | |
Description | Data deposited in or computed by PubChem | |
InChI |
InChI=1S/C42H77N7O28/c43-1-8-29-15(50)22(57)36(64-8)72-30-9(2-44)66-38(24(59)17(30)52)74-32-11(4-46)68-40(26(61)19(32)54)76-34-13(6-48)70-42(28(63)21(34)56)77-35-14(7-49)69-41(27(62)20(35)55)75-33-12(5-47)67-39(25(60)18(33)53)73-31-10(3-45)65-37(71-29)23(58)16(31)51/h8-42,50-63H,1-7,43-49H2/t8-,9-,10-,11-,12-,13-,14-,15-,16-,17-,18-,19-,20-,21-,22-,23-,24-,25-,26-,27-,28-,29-,30-,31-,32-,33-,34-,35-,36-,37-,38-,39-,40-,41-,42-/m1/s1 | |
Details | Computed by InChI 1.0.6 (PubChem release 2021.05.07) | |
Source | PubChem | |
URL | https://pubchem.ncbi.nlm.nih.gov | |
Description | Data deposited in or computed by PubChem | |
InChI Key |
GOPKBPURTDZYDJ-FOUAGVGXSA-N | |
Details | Computed by InChI 1.0.6 (PubChem release 2021.05.07) | |
Source | PubChem | |
URL | https://pubchem.ncbi.nlm.nih.gov | |
Description | Data deposited in or computed by PubChem | |
Canonical SMILES |
C(C1C2C(C(C(O1)OC3C(OC(C(C3O)O)OC4C(OC(C(C4O)O)OC5C(OC(C(C5O)O)OC6C(OC(C(C6O)O)OC7C(OC(C(C7O)O)OC8C(OC(O2)C(C8O)O)CN)CN)CN)CN)CN)CN)O)O)N | |
Details | Computed by OEChem 2.3.0 (PubChem release 2021.05.07) | |
Source | PubChem | |
URL | https://pubchem.ncbi.nlm.nih.gov | |
Description | Data deposited in or computed by PubChem | |
Isomeric SMILES |
C([C@@H]1[C@@H]2[C@@H]([C@H]([C@H](O1)O[C@@H]3[C@H](O[C@@H]([C@@H]([C@H]3O)O)O[C@@H]4[C@H](O[C@@H]([C@@H]([C@H]4O)O)O[C@@H]5[C@H](O[C@@H]([C@@H]([C@H]5O)O)O[C@@H]6[C@H](O[C@@H]([C@@H]([C@H]6O)O)O[C@@H]7[C@H](O[C@@H]([C@@H]([C@H]7O)O)O[C@@H]8[C@H](O[C@H](O2)[C@@H]([C@H]8O)O)CN)CN)CN)CN)CN)CN)O)O)N | |
Details | Computed by OEChem 2.3.0 (PubChem release 2021.05.07) | |
Source | PubChem | |
URL | https://pubchem.ncbi.nlm.nih.gov | |
Description | Data deposited in or computed by PubChem | |
Molecular Formula |
C42H77N7O28 | |
Details | Computed by PubChem 2.1 (PubChem release 2021.05.07) | |
Source | PubChem | |
URL | https://pubchem.ncbi.nlm.nih.gov | |
Description | Data deposited in or computed by PubChem | |
Molecular Weight |
1128.1 g/mol | |
Details | Computed by PubChem 2.1 (PubChem release 2021.05.07) | |
Source | PubChem | |
URL | https://pubchem.ncbi.nlm.nih.gov | |
Description | Data deposited in or computed by PubChem | |
Retrosynthesis Analysis
AI-Powered Synthesis Planning: Our tool employs the Template_relevance Pistachio, Template_relevance Bkms_metabolic, Template_relevance Pistachio_ringbreaker, Template_relevance Reaxys, Template_relevance Reaxys_biocatalysis model, leveraging a vast database of chemical reactions to predict feasible synthetic routes.
One-Step Synthesis Focus: Specifically designed for one-step synthesis, it provides concise and direct routes for your target compounds, streamlining the synthesis process.
Accurate Predictions: Utilizing the extensive PISTACHIO, BKMS_METABOLIC, PISTACHIO_RINGBREAKER, REAXYS, REAXYS_BIOCATALYSIS database, our tool offers high-accuracy predictions, reflecting the latest in chemical research and data.
Strategy Settings
Precursor scoring | Relevance Heuristic |
---|---|
Min. plausibility | 0.01 |
Model | Template_relevance |
Template Set | Pistachio/Bkms_metabolic/Pistachio_ringbreaker/Reaxys/Reaxys_biocatalysis |
Top-N result to add to graph | 6 |
Feasible Synthetic Routes
Q1: How does Per-6-amino-beta-cyclodextrin interact with its targets, and what are the downstream effects?
A: this compound (Per-6-ABCD) is a modified cyclodextrin with amine groups that allow it to interact with various molecules. Its primary interaction mode is through host-guest complexation, where the molecule acts as a host encapsulating guest molecules within its hydrophobic cavity [, , ]. This complexation can lead to various downstream effects depending on the guest molecule. For example:
- Enhanced solubility and bioavailability: Per-6-ABCD can encapsulate hydrophobic drug molecules, improving their solubility and bioavailability in aqueous solutions [].
- Controlled release: Complexation with Per-6-ABCD can modulate the release kinetics of encapsulated molecules, making it useful for drug delivery applications [].
- Catalysis: Per-6-ABCD can act as a supramolecular catalyst by bringing reactants into close proximity within its cavity, promoting specific reactions [].
Q2: What are the catalytic properties and applications of this compound?
A: this compound exhibits catalytic activity in various reactions, acting as a supramolecular catalyst. One example is its use in the N-arylation of imidazole with aryl bromides, catalyzed by copper(I) []. The Per-6-ABCD acts as both a supramolecular ligand for copper(I) and a host for the aryl bromides, bringing the reactants into close proximity within its hydrophobic cavity and promoting the reaction []. This method shows excellent yields and tolerates various functional groups, highlighting the potential of Per-6-ABCD in developing efficient and environmentally friendly catalytic processes [].
Q3: How does the structure of this compound influence its material compatibility and stability?
A3: this compound's structure significantly impacts its material compatibility and stability. The presence of amine groups allows for versatile modifications and interactions with other materials. For example:
- Graphene oxide hybrid: Per-6-ABCD can form hybrids with graphene oxide, creating supramolecular polymer networks upon the addition of guest molecules []. This hybrid material combines the properties of both components, offering potential applications in various fields, such as sensing and drug delivery.
- Electrode modification: Per-6-ABCD can be used to modify electrode surfaces, enhancing their sensitivity and selectivity for specific analytes []. For instance, a glassy carbon electrode modified with Per-6-ABCD and functionalized single-walled carbon nanotubes exhibited improved dopamine detection even in the presence of ascorbic acid [].
Q4: Can you provide insights into the structure-activity relationship (SAR) of this compound?
A4: While the provided research excerpts don't delve deep into specific SAR studies for Per-6-ABCD, they offer valuable insights:
- Importance of amine groups: The presence of amine groups is crucial for Per-6-ABCD's ability to interact with guest molecules, form hybrids with other materials like graphene oxide [], and act as a ligand for metal catalysts []. Modification of these amine groups could potentially alter these properties.
- Role of cavity size: The size of the hydrophobic cavity in Per-6-ABCD plays a vital role in its host-guest complexation abilities [, ]. Modifying the cyclodextrin ring size could impact the size and shape of the cavity, affecting its affinity for specific guest molecules.
- Surface modifications: Attaching functional groups to the Per-6-ABCD structure can alter its properties, as seen in its use for modifying electrode surfaces with carbon nanotubes []. This highlights the potential for tailoring its properties through targeted structural modifications.
Q5: What analytical methods and techniques are commonly employed to characterize and quantify this compound?
A5: Various analytical techniques are used to characterize this compound and its interactions with other molecules. The research excerpts mention the following methods:
- Nuclear Magnetic Resonance (NMR) spectroscopy: NMR can provide structural information about Per-6-ABCD and its complexes with guest molecules []. This technique is valuable for studying host-guest interactions and characterizing the binding modes of different molecules within the Per-6-ABCD cavity.
- Mass spectrometry (MS): MS is used to determine the molecular weight of Per-6-ABCD and its complexes, offering insights into the stoichiometry of interactions with other molecules [].
- Electrochemical techniques: Cyclic voltammetry, square wave voltammetry, and electrochemical impedance spectroscopy are employed to study the electrochemical behavior of Per-6-ABCD modified electrodes and their interactions with analytes like dopamine []. These techniques are particularly useful for evaluating the sensing capabilities of Per-6-ABCD based materials.
- Surface characterization techniques: Techniques like contact angle measurements, ellipsometry, Brewster angle Fourier transform infrared spectroscopy, X-ray photoelectron spectroscopy, and time-of-flight secondary ion mass spectrometry are used to characterize Per-6-ABCD monolayers on surfaces []. These methods provide information about the surface coverage, thickness, and chemical composition of the modified surfaces.
試験管内研究製品の免責事項と情報
BenchChemで提示されるすべての記事および製品情報は、情報提供を目的としています。BenchChemで購入可能な製品は、生体外研究のために特別に設計されています。生体外研究は、ラテン語の "in glass" に由来し、生物体の外で行われる実験を指します。これらの製品は医薬品または薬として分類されておらず、FDAから任何の医療状態、病気、または疾患の予防、治療、または治癒のために承認されていません。これらの製品を人間または動物に体内に導入する形態は、法律により厳格に禁止されています。これらのガイドラインに従うことは、研究と実験において法的および倫理的な基準の遵守を確実にするために重要です。