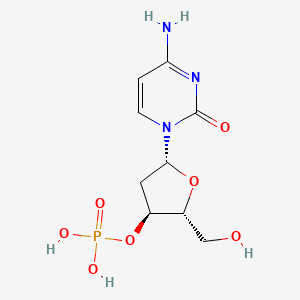
2'-デオキシシチジン-3'-モノリン酸
概要
説明
2’-Deoxycytidine-3’-monophosphate is a deoxynucleotide, which is one of the four monomers that make up DNA. It is a derivative of cytidine monophosphate, where the hydroxyl group at the 2’ position of the ribose sugar is replaced by a hydrogen atom, making it a deoxy sugar. This compound plays a crucial role in the formation and repair of DNA, and it pairs with deoxyguanosine monophosphate in the DNA double helix .
科学的研究の応用
2’-Deoxycytidine-3’-monophosphate has numerous applications in scientific research:
Chemistry: It is used as a model compound to study DNA strand breakage and nucleic acid base modifications.
Biology: It plays a role in DNA synthesis and repair studies.
Medicine: It is used in the development of antiviral and anticancer drugs, particularly in the study of nucleoside analogues.
Industry: It is used in the production of synthetic DNA and RNA for various biotechnological applications.
作用機序
Target of Action
The primary targets of 2’-Deoxycytidine-3’-monophosphate (dCMP) are enzymes such as cytidine deaminase (CDD) and deoxycytidine monophosphate deaminase (dCMP deaminase) . These enzymes play a crucial role in the metabolism of deoxycytidine analogues, which are widely used for the treatment of malignant diseases .
Mode of Action
dCMP interacts with its targets, CDD and dCMP deaminase, leading to their inactivation . This interaction can result in changes in the metabolic pathways of deoxycytidine analogues, affecting their activation or inactivation . The compound’s action can be influenced by other enzymes such as deoxycytidine kinase, which is important for the metabolism of deoxycytidine analogues .
Biochemical Pathways
dCMP affects several biochemical pathways. It is involved in the metabolism of deoxycytidine analogues, which can be inactivated by CDD or dCMP deaminase . Additional metabolic pathways, such as phosphorylation, can also contribute to their activation or inactivation . The compound’s action can be influenced by the aggregated impact of cytidine transporters, CDD, dCMP deaminase, and deoxycytidine kinase .
Pharmacokinetics
It is known that the compound’s action can be influenced by a wide range of mechanisms involving transport, phosphorylation, and catabolism .
Result of Action
The action of dCMP results in changes in the metabolism of deoxycytidine analogues, affecting their activation or inactivation . This can lead to changes in the therapeutic action of these analogues, which are widely used for the treatment of malignant diseases . For example, the key prerequisite of the therapeutic action of deoxycytidine analogues is their incorporation into DNA .
Action Environment
The action, efficacy, and stability of dCMP can be influenced by various environmental factors. These can include the presence of other enzymes and compounds in the cell, as well as factors related to the cell’s metabolic state . .
準備方法
Synthetic Routes and Reaction Conditions: The synthesis of 2’-Deoxycytidine-3’-monophosphate typically involves the phosphorylation of 2’-deoxycytidine. This can be achieved through various chemical or enzymatic methods. One common approach is the use of phosphorylating agents such as phosphorus oxychloride or phosphoramidite chemistry under controlled conditions to ensure the selective formation of the 3’-monophosphate .
Industrial Production Methods: Industrial production of 2’-Deoxycytidine-3’-monophosphate often involves the use of genetically engineered microorganisms. For instance, Escherichia coli strains can be metabolically engineered to enhance the production of deoxycytidine, which is then phosphorylated to form 2’-Deoxycytidine-3’-monophosphate. This method involves the deletion of specific degradation enzymes and the overexpression of biosynthetic pathway genes to increase yield .
化学反応の分析
Types of Reactions: 2’-Deoxycytidine-3’-monophosphate undergoes various chemical reactions, including:
Oxidation: It can be oxidized to form 2’-deoxyuridine-3’-monophosphate.
Reduction: Reduction reactions are less common but can occur under specific conditions.
Substitution: It can undergo nucleophilic substitution reactions, particularly at the phosphate group.
Common Reagents and Conditions:
Oxidation: Common oxidizing agents include potassium permanganate and hydrogen peroxide.
Substitution: Nucleophiles such as hydroxide ions or amines can be used under basic conditions.
Major Products:
Oxidation: 2’-Deoxyuridine-3’-monophosphate.
Substitution: Various substituted derivatives depending on the nucleophile used.
類似化合物との比較
- 2’-Deoxycytidine-5’-monophosphate
- 2’-Deoxyuridine-3’-monophosphate
- 2’-Deoxyadenosine-3’-monophosphate
Comparison: 2’-Deoxycytidine-3’-monophosphate is unique in its specific pairing with deoxyguanosine monophosphate in DNA. Unlike 2’-Deoxyuridine-3’-monophosphate, which pairs with deoxyadenosine monophosphate, 2’-Deoxycytidine-3’-monophosphate maintains the cytosine-guanine pairing essential for DNA stability. Additionally, its role in DNA synthesis and repair makes it distinct from other deoxynucleotides .
特性
IUPAC Name |
[5-(4-amino-2-oxopyrimidin-1-yl)-2-(hydroxymethyl)oxolan-3-yl] dihydrogen phosphate | |
---|---|---|
Details | Computed by Lexichem TK 2.7.0 (PubChem release 2021.10.14) | |
Source | PubChem | |
URL | https://pubchem.ncbi.nlm.nih.gov | |
Description | Data deposited in or computed by PubChem | |
InChI |
InChI=1S/C9H14N3O7P/c10-7-1-2-12(9(14)11-7)8-3-5(6(4-13)18-8)19-20(15,16)17/h1-2,5-6,8,13H,3-4H2,(H2,10,11,14)(H2,15,16,17) | |
Details | Computed by InChI 1.0.6 (PubChem release 2021.10.14) | |
Source | PubChem | |
URL | https://pubchem.ncbi.nlm.nih.gov | |
Description | Data deposited in or computed by PubChem | |
InChI Key |
FVSAHFFHPOLBLL-UHFFFAOYSA-N | |
Details | Computed by InChI 1.0.6 (PubChem release 2021.10.14) | |
Source | PubChem | |
URL | https://pubchem.ncbi.nlm.nih.gov | |
Description | Data deposited in or computed by PubChem | |
Canonical SMILES |
C1C(C(OC1N2C=CC(=NC2=O)N)CO)OP(=O)(O)O | |
Details | Computed by OEChem 2.3.0 (PubChem release 2021.10.14) | |
Source | PubChem | |
URL | https://pubchem.ncbi.nlm.nih.gov | |
Description | Data deposited in or computed by PubChem | |
Molecular Formula |
C9H14N3O7P | |
Details | Computed by PubChem 2.2 (PubChem release 2021.10.14) | |
Source | PubChem | |
URL | https://pubchem.ncbi.nlm.nih.gov | |
Description | Data deposited in or computed by PubChem | |
DSSTOX Substance ID |
DTXSID10390924 | |
Record name | AC1MNA3J | |
Source | EPA DSSTox | |
URL | https://comptox.epa.gov/dashboard/DTXSID10390924 | |
Description | DSSTox provides a high quality public chemistry resource for supporting improved predictive toxicology. | |
Molecular Weight |
307.20 g/mol | |
Details | Computed by PubChem 2.2 (PubChem release 2021.10.14) | |
Source | PubChem | |
URL | https://pubchem.ncbi.nlm.nih.gov | |
Description | Data deposited in or computed by PubChem | |
CAS No. |
6220-63-9 | |
Record name | AC1MNA3J | |
Source | EPA DSSTox | |
URL | https://comptox.epa.gov/dashboard/DTXSID10390924 | |
Description | DSSTox provides a high quality public chemistry resource for supporting improved predictive toxicology. | |
Retrosynthesis Analysis
AI-Powered Synthesis Planning: Our tool employs the Template_relevance Pistachio, Template_relevance Bkms_metabolic, Template_relevance Pistachio_ringbreaker, Template_relevance Reaxys, Template_relevance Reaxys_biocatalysis model, leveraging a vast database of chemical reactions to predict feasible synthetic routes.
One-Step Synthesis Focus: Specifically designed for one-step synthesis, it provides concise and direct routes for your target compounds, streamlining the synthesis process.
Accurate Predictions: Utilizing the extensive PISTACHIO, BKMS_METABOLIC, PISTACHIO_RINGBREAKER, REAXYS, REAXYS_BIOCATALYSIS database, our tool offers high-accuracy predictions, reflecting the latest in chemical research and data.
Strategy Settings
Precursor scoring | Relevance Heuristic |
---|---|
Min. plausibility | 0.01 |
Model | Template_relevance |
Template Set | Pistachio/Bkms_metabolic/Pistachio_ringbreaker/Reaxys/Reaxys_biocatalysis |
Top-N result to add to graph | 6 |
Feasible Synthetic Routes
Q1: How do low-energy electrons (LEEs) induce single-strand breaks (SSBs) in DNA, specifically involving 2'-Deoxycytidine-3'-monophosphate (3'-dCMP)?
A1: Research suggests that LEEs, with energies between 0-3 eV, can attach to 3'-dCMP, forming a transient negative ion [, ]. This electron often localizes on the cytosine base initially. Subsequently, the excess negative charge can migrate through overlapping atomic orbitals, primarily from the C6 carbon of the cytosine base to the C3' carbon of the ribose sugar []. This charge transfer weakens the C3'-O3' bond in the DNA backbone, making it susceptible to breakage, ultimately leading to a SSB [, ]. Theoretical calculations highlight that the energy barrier for C3'-O3' bond cleavage is significantly lower than that for other bonds like C5'-O5' or the N-glycosidic bond, making it the most probable site for LEE-induced SSB [].
Q2: What role does quantum tunneling play in LEE-induced single-strand breaks in 3'-dCMP?
A2: While charge transfer is crucial for initiating SSBs, quantum tunneling becomes particularly significant at higher LEE energies (above 1 eV) []. Theoretical studies employing time-dependent calculations show that at these energies, the 3'-dCMP anion can exist in vibrationally excited states []. These excited states increase the probability of the C3'-O3' bond tunneling through the energy barrier, even without overcoming it directly, leading to bond breaking and SSB formation []. This mechanism, different from the charge-induced dissociation observed at lower energies, highlights the complex interplay of factors influencing LEE-induced DNA damage.
Q3: Can 3'-dCMP capture very low energy electrons, and what are the implications?
A3: Yes, theoretical studies using density functional theory calculations suggest that 3'-dCMP can capture electrons with near-zero energy, both in a gaseous environment and in aqueous solutions []. This capture forms a radical anion, with the excess electron density primarily residing on the cytosine base []. The resulting radical anion is more stable than its neutral counterpart, and its electron detachment energy is substantial enough to enable subsequent bond breaking events in the DNA backbone, leading to strand breaks []. This finding emphasizes the potential for even very low energy electrons to initiate DNA damage.
Q4: How do analytical techniques contribute to our understanding of 3'-dCMP modifications?
A5: Several analytical techniques have proven valuable in studying 3'-dCMP modifications. For instance, 32P-postlabeling has been successfully employed to detect and characterize DNA adducts formed by the reaction of 3'-dCMP with styrene oxide, a known carcinogen []. This technique allows researchers to identify specific adducts and quantify their formation, shedding light on the mechanisms of DNA damage induced by various agents.
試験管内研究製品の免責事項と情報
BenchChemで提示されるすべての記事および製品情報は、情報提供を目的としています。BenchChemで購入可能な製品は、生体外研究のために特別に設計されています。生体外研究は、ラテン語の "in glass" に由来し、生物体の外で行われる実験を指します。これらの製品は医薬品または薬として分類されておらず、FDAから任何の医療状態、病気、または疾患の予防、治療、または治癒のために承認されていません。これらの製品を人間または動物に体内に導入する形態は、法律により厳格に禁止されています。これらのガイドラインに従うことは、研究と実験において法的および倫理的な基準の遵守を確実にするために重要です。