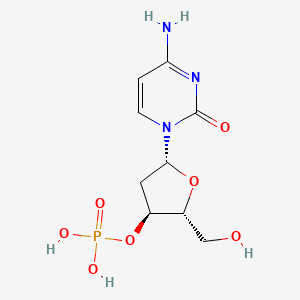
2'-Deoxycytidine-3'-monophosphate
Overview
Description
2’-Deoxycytidine-3’-monophosphate (3’-dCMP) is a nucleotide analog and a precursor to the synthesis of DNA. It is commonly used in the pharmaceutical industry as an antiviral agent, particularly for treating diseases caused by DNA viruses such as herpes and varicella-zoster . In a DNA double helix, it will base pair with deoxyguanosine monophosphate .
Molecular Structure Analysis
The chemical formula of 2’-deoxycytidine-3’-monophosphate is C9H14N3O7P . It consists of a deoxyribose sugar, a cytosine base, and a phosphate group. The molecular weight is approximately 307.2 g/mol .
Scientific Research Applications
DNA Synthesis Inhibition
2'-Deoxycytidine analogues, such as 2',2'-difluorodeoxycytidine, have been studied for their action on DNA synthesis. These compounds are known to inhibit DNA synthesis in a concentration-dependent manner and are incorporated into cellular DNA. They demonstrate distinct molecular actions on DNA metabolism, suggesting their potential use in understanding DNA replication and as tools in cancer research (Huang et al., 1991).
Radioactive Labeling in Biological Research
2'-Deoxycytidine-3'-monophosphate is used in the preparation of radioactive monophosphates for biological and biochemical experiments. This application is crucial for tracking and analyzing nucleotide behavior in various biological contexts (Vilpo & Vilpo, 1989).
Theoretical and Computational Studies
The molecule has been the subject of theoretical analysis to understand its protonation and electron density distribution. Such studies are vital for comprehending the molecular structure and behavior of nucleotides in different environments (Starikov & Pedash, 1990).
Structural Analysis and Conformation Studies
Infrared spectroscopy and theoretical calculations have been used to explore the gas phase structures of deprotonated 2'-deoxymononucleotides. These studies help in understanding the conformations and energetics of nucleotides, which is crucial for designing drugs and studying DNA-protein interactions (Nei et al., 2013).
Biosynthesis and Application in Medicine
This compound is used in the biosynthesis of deoxynucleoside-5'-monophosphates, which are basic components of DNA and are used widely in medicine and as biochemical reagents (Zou et al., 2013).
Chromatographic Analysis in DNA Methylation Studies
High-performance liquid chromatography has been optimized for separating 2'-deoxycytidine-5'-monophosphate and its variants. This method is essential for determining the methylation state of DNA, a critical factor in genetic regulation and disease studies (Havliš et al., 2001).
Prodrug Approach in Cellular Delivery
Studies have explored using 2'-deoxycytidine analogues for cellular delivery and generation of nucleoside diphosphates, which can be potent inhibitors of ribonucleotide reductase. This approach has potential applications in the treatment of various diseases, including AIDS (Kang et al., 1997).
Mechanism of Action
Target of Action
The primary targets of 2’-Deoxycytidine-3’-monophosphate (dCMP) are enzymes such as cytidine deaminase (CDD) and deoxycytidine monophosphate deaminase (dCMP deaminase) . These enzymes play a crucial role in the metabolism of deoxycytidine analogues, which are widely used for the treatment of malignant diseases .
Mode of Action
dCMP interacts with its targets, CDD and dCMP deaminase, leading to their inactivation . This interaction can result in changes in the metabolic pathways of deoxycytidine analogues, affecting their activation or inactivation . The compound’s action can be influenced by other enzymes such as deoxycytidine kinase, which is important for the metabolism of deoxycytidine analogues .
Biochemical Pathways
dCMP affects several biochemical pathways. It is involved in the metabolism of deoxycytidine analogues, which can be inactivated by CDD or dCMP deaminase . Additional metabolic pathways, such as phosphorylation, can also contribute to their activation or inactivation . The compound’s action can be influenced by the aggregated impact of cytidine transporters, CDD, dCMP deaminase, and deoxycytidine kinase .
Pharmacokinetics
It is known that the compound’s action can be influenced by a wide range of mechanisms involving transport, phosphorylation, and catabolism .
Result of Action
The action of dCMP results in changes in the metabolism of deoxycytidine analogues, affecting their activation or inactivation . This can lead to changes in the therapeutic action of these analogues, which are widely used for the treatment of malignant diseases . For example, the key prerequisite of the therapeutic action of deoxycytidine analogues is their incorporation into DNA .
Action Environment
The action, efficacy, and stability of dCMP can be influenced by various environmental factors. These can include the presence of other enzymes and compounds in the cell, as well as factors related to the cell’s metabolic state . .
Properties
IUPAC Name |
[5-(4-amino-2-oxopyrimidin-1-yl)-2-(hydroxymethyl)oxolan-3-yl] dihydrogen phosphate | |
---|---|---|
Details | Computed by Lexichem TK 2.7.0 (PubChem release 2021.10.14) | |
Source | PubChem | |
URL | https://pubchem.ncbi.nlm.nih.gov | |
Description | Data deposited in or computed by PubChem | |
InChI |
InChI=1S/C9H14N3O7P/c10-7-1-2-12(9(14)11-7)8-3-5(6(4-13)18-8)19-20(15,16)17/h1-2,5-6,8,13H,3-4H2,(H2,10,11,14)(H2,15,16,17) | |
Details | Computed by InChI 1.0.6 (PubChem release 2021.10.14) | |
Source | PubChem | |
URL | https://pubchem.ncbi.nlm.nih.gov | |
Description | Data deposited in or computed by PubChem | |
InChI Key |
FVSAHFFHPOLBLL-UHFFFAOYSA-N | |
Details | Computed by InChI 1.0.6 (PubChem release 2021.10.14) | |
Source | PubChem | |
URL | https://pubchem.ncbi.nlm.nih.gov | |
Description | Data deposited in or computed by PubChem | |
Canonical SMILES |
C1C(C(OC1N2C=CC(=NC2=O)N)CO)OP(=O)(O)O | |
Details | Computed by OEChem 2.3.0 (PubChem release 2021.10.14) | |
Source | PubChem | |
URL | https://pubchem.ncbi.nlm.nih.gov | |
Description | Data deposited in or computed by PubChem | |
Molecular Formula |
C9H14N3O7P | |
Details | Computed by PubChem 2.2 (PubChem release 2021.10.14) | |
Source | PubChem | |
URL | https://pubchem.ncbi.nlm.nih.gov | |
Description | Data deposited in or computed by PubChem | |
DSSTOX Substance ID |
DTXSID10390924 | |
Record name | AC1MNA3J | |
Source | EPA DSSTox | |
URL | https://comptox.epa.gov/dashboard/DTXSID10390924 | |
Description | DSSTox provides a high quality public chemistry resource for supporting improved predictive toxicology. | |
Molecular Weight |
307.20 g/mol | |
Details | Computed by PubChem 2.2 (PubChem release 2021.10.14) | |
Source | PubChem | |
URL | https://pubchem.ncbi.nlm.nih.gov | |
Description | Data deposited in or computed by PubChem | |
CAS No. |
6220-63-9 | |
Record name | AC1MNA3J | |
Source | EPA DSSTox | |
URL | https://comptox.epa.gov/dashboard/DTXSID10390924 | |
Description | DSSTox provides a high quality public chemistry resource for supporting improved predictive toxicology. | |
Retrosynthesis Analysis
AI-Powered Synthesis Planning: Our tool employs the Template_relevance Pistachio, Template_relevance Bkms_metabolic, Template_relevance Pistachio_ringbreaker, Template_relevance Reaxys, Template_relevance Reaxys_biocatalysis model, leveraging a vast database of chemical reactions to predict feasible synthetic routes.
One-Step Synthesis Focus: Specifically designed for one-step synthesis, it provides concise and direct routes for your target compounds, streamlining the synthesis process.
Accurate Predictions: Utilizing the extensive PISTACHIO, BKMS_METABOLIC, PISTACHIO_RINGBREAKER, REAXYS, REAXYS_BIOCATALYSIS database, our tool offers high-accuracy predictions, reflecting the latest in chemical research and data.
Strategy Settings
Precursor scoring | Relevance Heuristic |
---|---|
Min. plausibility | 0.01 |
Model | Template_relevance |
Template Set | Pistachio/Bkms_metabolic/Pistachio_ringbreaker/Reaxys/Reaxys_biocatalysis |
Top-N result to add to graph | 6 |
Feasible Synthetic Routes
Q1: How do low-energy electrons (LEEs) induce single-strand breaks (SSBs) in DNA, specifically involving 2'-Deoxycytidine-3'-monophosphate (3'-dCMP)?
A1: Research suggests that LEEs, with energies between 0-3 eV, can attach to 3'-dCMP, forming a transient negative ion [, ]. This electron often localizes on the cytosine base initially. Subsequently, the excess negative charge can migrate through overlapping atomic orbitals, primarily from the C6 carbon of the cytosine base to the C3' carbon of the ribose sugar []. This charge transfer weakens the C3'-O3' bond in the DNA backbone, making it susceptible to breakage, ultimately leading to a SSB [, ]. Theoretical calculations highlight that the energy barrier for C3'-O3' bond cleavage is significantly lower than that for other bonds like C5'-O5' or the N-glycosidic bond, making it the most probable site for LEE-induced SSB [].
Q2: What role does quantum tunneling play in LEE-induced single-strand breaks in 3'-dCMP?
A2: While charge transfer is crucial for initiating SSBs, quantum tunneling becomes particularly significant at higher LEE energies (above 1 eV) []. Theoretical studies employing time-dependent calculations show that at these energies, the 3'-dCMP anion can exist in vibrationally excited states []. These excited states increase the probability of the C3'-O3' bond tunneling through the energy barrier, even without overcoming it directly, leading to bond breaking and SSB formation []. This mechanism, different from the charge-induced dissociation observed at lower energies, highlights the complex interplay of factors influencing LEE-induced DNA damage.
Q3: Can 3'-dCMP capture very low energy electrons, and what are the implications?
A3: Yes, theoretical studies using density functional theory calculations suggest that 3'-dCMP can capture electrons with near-zero energy, both in a gaseous environment and in aqueous solutions []. This capture forms a radical anion, with the excess electron density primarily residing on the cytosine base []. The resulting radical anion is more stable than its neutral counterpart, and its electron detachment energy is substantial enough to enable subsequent bond breaking events in the DNA backbone, leading to strand breaks []. This finding emphasizes the potential for even very low energy electrons to initiate DNA damage.
Q4: How do analytical techniques contribute to our understanding of 3'-dCMP modifications?
A5: Several analytical techniques have proven valuable in studying 3'-dCMP modifications. For instance, 32P-postlabeling has been successfully employed to detect and characterize DNA adducts formed by the reaction of 3'-dCMP with styrene oxide, a known carcinogen []. This technique allows researchers to identify specific adducts and quantify their formation, shedding light on the mechanisms of DNA damage induced by various agents.
Disclaimer and Information on In-Vitro Research Products
Please be aware that all articles and product information presented on BenchChem are intended solely for informational purposes. The products available for purchase on BenchChem are specifically designed for in-vitro studies, which are conducted outside of living organisms. In-vitro studies, derived from the Latin term "in glass," involve experiments performed in controlled laboratory settings using cells or tissues. It is important to note that these products are not categorized as medicines or drugs, and they have not received approval from the FDA for the prevention, treatment, or cure of any medical condition, ailment, or disease. We must emphasize that any form of bodily introduction of these products into humans or animals is strictly prohibited by law. It is essential to adhere to these guidelines to ensure compliance with legal and ethical standards in research and experimentation.