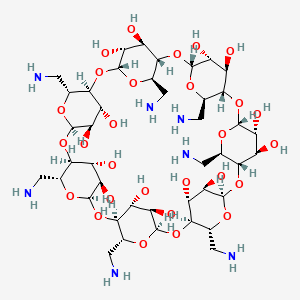
Per-6-amino-beta-cyclodextrin
Vue d'ensemble
Description
Per-6-amino-beta-cyclodextrin is a derivative of beta-cyclodextrin, a cyclic oligosaccharide composed of seven glucose units linked by alpha-1,4-glycosidic bonds. This compound is characterized by the substitution of amino groups at the primary hydroxyl positions of the beta-cyclodextrin molecule. The unique structure of this compound allows it to form inclusion complexes with various guest molecules, making it valuable in numerous scientific and industrial applications .
Mécanisme D'action
Target of Action
Per-6-amino-beta-cyclodextrin (PA-β-CD) primarily targets aryl halides and imidazole . It acts as a supramolecular ligand for CuI and a host for aryl bromides .
Mode of Action
PA-β-CD interacts with its targets to catalyze specific reactions. For instance, it catalyzes the N-arylation of imidazole with aryl bromides under mild conditions . It also promotes the one-pot synthesis of enantiomerically-enriched 2-aryl-2,3-dihydroquinolin-4(1H)-ones .
Biochemical Pathways
PA-β-CD affects the cyanation of aryl halides . It uses less toxic K4 [Fe (CN)6] as the reagent and amino-β-cyclodextrins as supramolecular ligands for CuI . This leads to the production of aryl and heteroaryl nitriles .
Result of Action
The action of PA-β-CD results in the synthesis of enantiomerically-enriched 2-aryl-2,3-dihydroquinolin-4(1H)-ones with high yield (up to 99%) and enantiomeric excess (up to 99%) . It also leads to the efficient cyanation of aryl halides .
Action Environment
The action of PA-β-CD is influenced by environmental factors. For instance, it can catalyze reactions under mild conditions . , suggesting that they can function effectively in aqueous environments.
Analyse Biochimique
Biochemical Properties
Per-6-amino-beta-cyclodextrin plays a crucial role in biochemical reactions, primarily as a supramolecular ligand and host. It interacts with various enzymes, proteins, and other biomolecules, facilitating catalytic processes and enhancing reaction efficiency. For instance, this compound acts as a supramolecular ligand for copper(I) ions, catalyzing the N-arylation of imidazole with aryl bromides under mild conditions . This interaction demonstrates the compound’s ability to stabilize transition states and improve reaction yields. Additionally, this compound forms inclusion complexes with hydrophobic molecules, thereby enhancing their solubility and bioavailability .
Cellular Effects
This compound influences various cellular processes, including cell signaling pathways, gene expression, and cellular metabolism. It has been observed to interact with cell membranes, altering their cholesterol content and affecting membrane fluidity . This interaction can disrupt the barrier function of cell layers, leading to changes in cellular permeability and transport. Furthermore, this compound has been shown to modulate gene expression by forming complexes with transcription factors and other regulatory proteins, thereby influencing cellular responses to external stimuli .
Molecular Mechanism
The molecular mechanism of this compound involves its ability to form stable inclusion complexes with various biomolecules. This compound acts as a catalyst in several organic reactions by providing a suitable microenvironment for the reactants. For example, this compound catalyzes the synthesis of 2-aryl-2,3-dihydro-4-quinolones by interacting with benzaldehyde and o-aminoacetophenone . The formation of hydrogen bonds and donor-acceptor interactions within the cyclodextrin cavity enhances the reaction’s selectivity and yield. Additionally, this compound can inhibit or activate enzymes by binding to their active sites, thereby modulating their activity .
Temporal Effects in Laboratory Settings
In laboratory settings, the effects of this compound can change over time due to its stability and degradation properties. Studies have shown that this compound remains stable under various conditions, maintaining its catalytic activity over extended periods . Prolonged exposure to certain environmental factors, such as high temperatures or extreme pH levels, can lead to its degradation and reduced efficacy. Long-term studies have also indicated that this compound can have lasting effects on cellular function, including sustained changes in gene expression and metabolic activity .
Dosage Effects in Animal Models
The effects of this compound vary with different dosages in animal models. At lower doses, the compound has been shown to enhance the bioavailability and efficacy of co-administered drugs by forming inclusion complexes . At higher doses, this compound can exhibit toxic or adverse effects, such as disrupting cellular membranes and causing cytotoxicity . Threshold effects have been observed, where the compound’s beneficial properties are maximized at optimal dosages, while excessive amounts lead to detrimental outcomes.
Metabolic Pathways
This compound is involved in various metabolic pathways, interacting with enzymes and cofactors to modulate metabolic flux and metabolite levels. The compound has been shown to participate in the metabolism of hydrophobic molecules by forming inclusion complexes that facilitate their transport and degradation . Additionally, this compound can influence the activity of metabolic enzymes by binding to their active sites, thereby altering their catalytic efficiency and substrate specificity .
Transport and Distribution
Within cells and tissues, this compound is transported and distributed through interactions with specific transporters and binding proteins. The compound’s hydrophilic exterior and hydrophobic cavity enable it to traverse cellular membranes and accumulate in target tissues . This compound can also interact with membrane-bound receptors and transporters, facilitating its uptake and distribution within the cell . These interactions play a crucial role in determining the compound’s localization and bioavailability.
Subcellular Localization
This compound exhibits specific subcellular localization, which influences its activity and function. The compound is often directed to particular cellular compartments or organelles through targeting signals or post-translational modifications . For instance, this compound can localize to the endoplasmic reticulum or mitochondria, where it interacts with resident proteins and modulates their function . This targeted localization enhances the compound’s efficacy in catalyzing biochemical reactions and regulating cellular processes.
Méthodes De Préparation
Synthetic Routes and Reaction Conditions
The synthesis of per-6-amino-beta-cyclodextrin typically involves the modification of beta-cyclodextrin at the primary hydroxyl groups. One common method includes the monotosylation of beta-cyclodextrin, followed by azidation and reduction to introduce amino groups. This process can be optimized under continuous flow conditions to enhance efficiency and yield . Another approach involves the direct reaction of beta-cyclodextrin with liquid amines under heating conditions .
Industrial Production Methods
Industrial production of this compound may utilize similar synthetic routes but on a larger scale. Continuous flow synthesis is particularly advantageous for industrial applications due to its scalability and ability to maintain consistent reaction conditions .
Analyse Des Réactions Chimiques
Types of Reactions
Per-6-amino-beta-cyclodextrin undergoes various chemical reactions, including substitution, oxidation, and reduction. The amino groups at the primary positions make it reactive towards electrophiles, allowing for further functionalization .
Common Reagents and Conditions
Common reagents used in the reactions of this compound include sodium azide, triphenylphosphine, and carbon tetrabromide for azidation, followed by reduction agents such as hydrogen or lithium aluminum hydride . These reactions are typically carried out under controlled temperature and pressure conditions to ensure high yield and selectivity .
Major Products
The major products formed from the reactions of this compound depend on the specific reagents and conditions used. For example, reaction with benzaldehyde can yield inclusion complexes that enhance the selectivity and yield of 2-aryl-2,3-dihydro-4-quinolones .
Applications De Recherche Scientifique
Per-6-amino-beta-cyclodextrin has a wide range of applications in scientific research:
Comparaison Avec Des Composés Similaires
Similar Compounds
Similar compounds to per-6-amino-beta-cyclodextrin include other cyclodextrin derivatives such as:
Alpha-cyclodextrin: Composed of six glucose units.
Gamma-cyclodextrin: Composed of eight glucose units.
Per-6-azido-beta-cyclodextrin: A precursor to this compound.
Uniqueness
This compound is unique due to the presence of amino groups at all primary hydroxyl positions, which enhances its reactivity and ability to form stable inclusion complexes. This makes it particularly valuable in applications requiring high selectivity and efficiency .
Propriétés
IUPAC Name |
(1S,3R,5R,6S,8R,10R,11S,13R,15R,16S,18R,20R,21S,23R,25R,26S,28R,30R,31S,33R,35R,36R,37R,38R,39R,40R,41R,42R,43R,44R,45R,46R,47R,48R,49R)-5,10,15,20,25,30,35-heptakis(aminomethyl)-2,4,7,9,12,14,17,19,22,24,27,29,32,34-tetradecaoxaoctacyclo[31.2.2.23,6.28,11.213,16.218,21.223,26.228,31]nonatetracontane-36,37,38,39,40,41,42,43,44,45,46,47,48,49-tetradecol | |
---|---|---|
Details | Computed by Lexichem TK 2.7.0 (PubChem release 2021.05.07) | |
Source | PubChem | |
URL | https://pubchem.ncbi.nlm.nih.gov | |
Description | Data deposited in or computed by PubChem | |
InChI |
InChI=1S/C42H77N7O28/c43-1-8-29-15(50)22(57)36(64-8)72-30-9(2-44)66-38(24(59)17(30)52)74-32-11(4-46)68-40(26(61)19(32)54)76-34-13(6-48)70-42(28(63)21(34)56)77-35-14(7-49)69-41(27(62)20(35)55)75-33-12(5-47)67-39(25(60)18(33)53)73-31-10(3-45)65-37(71-29)23(58)16(31)51/h8-42,50-63H,1-7,43-49H2/t8-,9-,10-,11-,12-,13-,14-,15-,16-,17-,18-,19-,20-,21-,22-,23-,24-,25-,26-,27-,28-,29-,30-,31-,32-,33-,34-,35-,36-,37-,38-,39-,40-,41-,42-/m1/s1 | |
Details | Computed by InChI 1.0.6 (PubChem release 2021.05.07) | |
Source | PubChem | |
URL | https://pubchem.ncbi.nlm.nih.gov | |
Description | Data deposited in or computed by PubChem | |
InChI Key |
GOPKBPURTDZYDJ-FOUAGVGXSA-N | |
Details | Computed by InChI 1.0.6 (PubChem release 2021.05.07) | |
Source | PubChem | |
URL | https://pubchem.ncbi.nlm.nih.gov | |
Description | Data deposited in or computed by PubChem | |
Canonical SMILES |
C(C1C2C(C(C(O1)OC3C(OC(C(C3O)O)OC4C(OC(C(C4O)O)OC5C(OC(C(C5O)O)OC6C(OC(C(C6O)O)OC7C(OC(C(C7O)O)OC8C(OC(O2)C(C8O)O)CN)CN)CN)CN)CN)CN)O)O)N | |
Details | Computed by OEChem 2.3.0 (PubChem release 2021.05.07) | |
Source | PubChem | |
URL | https://pubchem.ncbi.nlm.nih.gov | |
Description | Data deposited in or computed by PubChem | |
Isomeric SMILES |
C([C@@H]1[C@@H]2[C@@H]([C@H]([C@H](O1)O[C@@H]3[C@H](O[C@@H]([C@@H]([C@H]3O)O)O[C@@H]4[C@H](O[C@@H]([C@@H]([C@H]4O)O)O[C@@H]5[C@H](O[C@@H]([C@@H]([C@H]5O)O)O[C@@H]6[C@H](O[C@@H]([C@@H]([C@H]6O)O)O[C@@H]7[C@H](O[C@@H]([C@@H]([C@H]7O)O)O[C@@H]8[C@H](O[C@H](O2)[C@@H]([C@H]8O)O)CN)CN)CN)CN)CN)CN)O)O)N | |
Details | Computed by OEChem 2.3.0 (PubChem release 2021.05.07) | |
Source | PubChem | |
URL | https://pubchem.ncbi.nlm.nih.gov | |
Description | Data deposited in or computed by PubChem | |
Molecular Formula |
C42H77N7O28 | |
Details | Computed by PubChem 2.1 (PubChem release 2021.05.07) | |
Source | PubChem | |
URL | https://pubchem.ncbi.nlm.nih.gov | |
Description | Data deposited in or computed by PubChem | |
Molecular Weight |
1128.1 g/mol | |
Details | Computed by PubChem 2.1 (PubChem release 2021.05.07) | |
Source | PubChem | |
URL | https://pubchem.ncbi.nlm.nih.gov | |
Description | Data deposited in or computed by PubChem | |
Retrosynthesis Analysis
AI-Powered Synthesis Planning: Our tool employs the Template_relevance Pistachio, Template_relevance Bkms_metabolic, Template_relevance Pistachio_ringbreaker, Template_relevance Reaxys, Template_relevance Reaxys_biocatalysis model, leveraging a vast database of chemical reactions to predict feasible synthetic routes.
One-Step Synthesis Focus: Specifically designed for one-step synthesis, it provides concise and direct routes for your target compounds, streamlining the synthesis process.
Accurate Predictions: Utilizing the extensive PISTACHIO, BKMS_METABOLIC, PISTACHIO_RINGBREAKER, REAXYS, REAXYS_BIOCATALYSIS database, our tool offers high-accuracy predictions, reflecting the latest in chemical research and data.
Strategy Settings
Precursor scoring | Relevance Heuristic |
---|---|
Min. plausibility | 0.01 |
Model | Template_relevance |
Template Set | Pistachio/Bkms_metabolic/Pistachio_ringbreaker/Reaxys/Reaxys_biocatalysis |
Top-N result to add to graph | 6 |
Feasible Synthetic Routes
Q1: How does Per-6-amino-beta-cyclodextrin interact with its targets, and what are the downstream effects?
A: this compound (Per-6-ABCD) is a modified cyclodextrin with amine groups that allow it to interact with various molecules. Its primary interaction mode is through host-guest complexation, where the molecule acts as a host encapsulating guest molecules within its hydrophobic cavity [, , ]. This complexation can lead to various downstream effects depending on the guest molecule. For example:
- Enhanced solubility and bioavailability: Per-6-ABCD can encapsulate hydrophobic drug molecules, improving their solubility and bioavailability in aqueous solutions [].
- Controlled release: Complexation with Per-6-ABCD can modulate the release kinetics of encapsulated molecules, making it useful for drug delivery applications [].
- Catalysis: Per-6-ABCD can act as a supramolecular catalyst by bringing reactants into close proximity within its cavity, promoting specific reactions [].
Q2: What are the catalytic properties and applications of this compound?
A: this compound exhibits catalytic activity in various reactions, acting as a supramolecular catalyst. One example is its use in the N-arylation of imidazole with aryl bromides, catalyzed by copper(I) []. The Per-6-ABCD acts as both a supramolecular ligand for copper(I) and a host for the aryl bromides, bringing the reactants into close proximity within its hydrophobic cavity and promoting the reaction []. This method shows excellent yields and tolerates various functional groups, highlighting the potential of Per-6-ABCD in developing efficient and environmentally friendly catalytic processes [].
Q3: How does the structure of this compound influence its material compatibility and stability?
A3: this compound's structure significantly impacts its material compatibility and stability. The presence of amine groups allows for versatile modifications and interactions with other materials. For example:
- Graphene oxide hybrid: Per-6-ABCD can form hybrids with graphene oxide, creating supramolecular polymer networks upon the addition of guest molecules []. This hybrid material combines the properties of both components, offering potential applications in various fields, such as sensing and drug delivery.
- Electrode modification: Per-6-ABCD can be used to modify electrode surfaces, enhancing their sensitivity and selectivity for specific analytes []. For instance, a glassy carbon electrode modified with Per-6-ABCD and functionalized single-walled carbon nanotubes exhibited improved dopamine detection even in the presence of ascorbic acid [].
Q4: Can you provide insights into the structure-activity relationship (SAR) of this compound?
A4: While the provided research excerpts don't delve deep into specific SAR studies for Per-6-ABCD, they offer valuable insights:
- Importance of amine groups: The presence of amine groups is crucial for Per-6-ABCD's ability to interact with guest molecules, form hybrids with other materials like graphene oxide [], and act as a ligand for metal catalysts []. Modification of these amine groups could potentially alter these properties.
- Role of cavity size: The size of the hydrophobic cavity in Per-6-ABCD plays a vital role in its host-guest complexation abilities [, ]. Modifying the cyclodextrin ring size could impact the size and shape of the cavity, affecting its affinity for specific guest molecules.
- Surface modifications: Attaching functional groups to the Per-6-ABCD structure can alter its properties, as seen in its use for modifying electrode surfaces with carbon nanotubes []. This highlights the potential for tailoring its properties through targeted structural modifications.
Q5: What analytical methods and techniques are commonly employed to characterize and quantify this compound?
A5: Various analytical techniques are used to characterize this compound and its interactions with other molecules. The research excerpts mention the following methods:
- Nuclear Magnetic Resonance (NMR) spectroscopy: NMR can provide structural information about Per-6-ABCD and its complexes with guest molecules []. This technique is valuable for studying host-guest interactions and characterizing the binding modes of different molecules within the Per-6-ABCD cavity.
- Mass spectrometry (MS): MS is used to determine the molecular weight of Per-6-ABCD and its complexes, offering insights into the stoichiometry of interactions with other molecules [].
- Electrochemical techniques: Cyclic voltammetry, square wave voltammetry, and electrochemical impedance spectroscopy are employed to study the electrochemical behavior of Per-6-ABCD modified electrodes and their interactions with analytes like dopamine []. These techniques are particularly useful for evaluating the sensing capabilities of Per-6-ABCD based materials.
- Surface characterization techniques: Techniques like contact angle measurements, ellipsometry, Brewster angle Fourier transform infrared spectroscopy, X-ray photoelectron spectroscopy, and time-of-flight secondary ion mass spectrometry are used to characterize Per-6-ABCD monolayers on surfaces []. These methods provide information about the surface coverage, thickness, and chemical composition of the modified surfaces.
Avertissement et informations sur les produits de recherche in vitro
Veuillez noter que tous les articles et informations sur les produits présentés sur BenchChem sont destinés uniquement à des fins informatives. Les produits disponibles à l'achat sur BenchChem sont spécifiquement conçus pour des études in vitro, qui sont réalisées en dehors des organismes vivants. Les études in vitro, dérivées du terme latin "in verre", impliquent des expériences réalisées dans des environnements de laboratoire contrôlés à l'aide de cellules ou de tissus. Il est important de noter que ces produits ne sont pas classés comme médicaments et n'ont pas reçu l'approbation de la FDA pour la prévention, le traitement ou la guérison de toute condition médicale, affection ou maladie. Nous devons souligner que toute forme d'introduction corporelle de ces produits chez les humains ou les animaux est strictement interdite par la loi. Il est essentiel de respecter ces directives pour assurer la conformité aux normes légales et éthiques en matière de recherche et d'expérimentation.