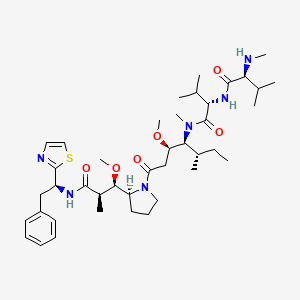
Mmad
Descripción general
Descripción
Desmetil-dolastatina 10, también conocida como Monometil Dolastatina 10, es un potente inhibidor de la tubulina derivado del producto natural marino Dolastatina 10. Es un análogo sintético de la Dolastatina 10, que fue originalmente aislada del molusco del Océano Índico Dolabella auricularia. La Desmetil-dolastatina 10 ha demostrado una actividad antitumoral significativa y se utiliza como carga tóxica en conjugados de anticuerpo-fármaco para la terapia dirigida del cáncer .
Aplicaciones Científicas De Investigación
La Desmetil-dolastatina 10 tiene una amplia gama de aplicaciones de investigación científica, incluyendo:
Química: Se utiliza como bloque de construcción en la síntesis de moléculas complejas y como reactivo en varias reacciones químicas.
Biología: Se estudia por sus efectos en los procesos celulares, particularmente su capacidad para inhibir la polimerización de la tubulina.
Medicina: Se utiliza en el desarrollo de conjugados de anticuerpo-fármaco para la terapia dirigida del cáncer. .
Industria: Se emplea en la producción de conjugados de anticuerpo-fármaco para aplicaciones farmacéuticas
Mecanismo De Acción
La Desmetil-dolastatina 10 ejerce sus efectos inhibiendo la polimerización de la tubulina, lo que interrumpe la formación de microtúbulos. Esta inhibición conduce al arresto del ciclo celular y la apoptosis en las células cancerosas. Los objetivos moleculares de la Desmetil-dolastatina 10 incluyen la tubulina, y afecta las vías implicadas en la división celular y la apoptosis .
Métodos De Preparación
Rutas sintéticas y condiciones de reacción
La síntesis de la Desmetil-dolastatina 10 implica múltiples pasos, incluyendo el acoplamiento de varios residuos de aminoácidos. Uno de los pasos clave es el proceso de ligación estable de la oxima, que produce conjugados de anticuerpo-fármaco casi homogéneos con una proporción de fármaco a anticuerpo de aproximadamente 2.0 . La solubilidad de este compuesto en dimetilsulfóxido es mayor que 10 milimolar. Para obtener una concentración más alta, el compuesto se puede calentar a 37 °C durante 10 minutos y/o agitar en un baño ultrasónico .
Métodos de producción industrial
La producción industrial de la Desmetil-dolastatina 10 normalmente implica la síntesis a gran escala utilizando las mismas rutas sintéticas que se describen anteriormente. El proceso garantiza una alta pureza y consistencia, lo que es crucial para su uso en conjugados de anticuerpo-fármaco .
Análisis De Reacciones Químicas
Tipos de reacciones
La Desmetil-dolastatina 10 se somete a varias reacciones químicas, incluyendo:
Oxidación: Esta reacción implica la adición de oxígeno o la eliminación de hidrógeno.
Reducción: Esta reacción implica la adición de hidrógeno o la eliminación de oxígeno.
Sustitución: Esta reacción implica la sustitución de un átomo o grupo de átomos por otro.
Reactivos y condiciones comunes
Los reactivos comunes utilizados en estas reacciones incluyen agentes oxidantes como el permanganato de potasio, agentes reductores como el borohidruro de sodio y varios nucleófilos para reacciones de sustitución. Las condiciones para estas reacciones varían dependiendo del producto deseado y la reacción específica que se esté realizando .
Principales productos formados
Los principales productos formados a partir de estas reacciones incluyen varios derivados de la Desmetil-dolastatina 10, que se pueden utilizar en modificaciones químicas adicionales o como intermediarios en la síntesis de moléculas más complejas .
Comparación Con Compuestos Similares
La Desmetil-dolastatina 10 es similar a otros inhibidores de la tubulina, como:
Dolastatina 10: El compuesto original del que se deriva la Desmetil-dolastatina 10. .
Monometil Auristatina E: Otro análogo sintético de la Dolastatina 10, utilizado en conjugados de anticuerpo-fármaco para la terapia del cáncer
Vinblastina: Un producto natural que inhibe la polimerización de la tubulina y se utiliza en la quimioterapia del cáncer.
La singularidad de la Desmetil-dolastatina 10 radica en sus modificaciones específicas, que mejoran su estabilidad y eficacia en los conjugados de anticuerpo-fármaco, lo que la convierte en una herramienta valiosa en la terapia dirigida del cáncer .
Propiedades
IUPAC Name |
(2S)-N-[(2S)-1-[[(3R,4S,5S)-3-methoxy-1-[(2S)-2-[(1R,2R)-1-methoxy-2-methyl-3-oxo-3-[[(1S)-2-phenyl-1-(1,3-thiazol-2-yl)ethyl]amino]propyl]pyrrolidin-1-yl]-5-methyl-1-oxoheptan-4-yl]-methylamino]-3-methyl-1-oxobutan-2-yl]-3-methyl-2-(methylamino)butanamide | |
---|---|---|
Source | PubChem | |
URL | https://pubchem.ncbi.nlm.nih.gov | |
Description | Data deposited in or computed by PubChem | |
InChI |
InChI=1S/C41H66N6O6S/c1-12-27(6)36(46(9)41(51)35(26(4)5)45-39(50)34(42-8)25(2)3)32(52-10)24-33(48)47-21-16-19-31(47)37(53-11)28(7)38(49)44-30(40-43-20-22-54-40)23-29-17-14-13-15-18-29/h13-15,17-18,20,22,25-28,30-32,34-37,42H,12,16,19,21,23-24H2,1-11H3,(H,44,49)(H,45,50)/t27-,28+,30-,31-,32+,34-,35-,36-,37+/m0/s1 | |
Source | PubChem | |
URL | https://pubchem.ncbi.nlm.nih.gov | |
Description | Data deposited in or computed by PubChem | |
InChI Key |
BLUGYPPOFIHFJS-UUFHNPECSA-N | |
Source | PubChem | |
URL | https://pubchem.ncbi.nlm.nih.gov | |
Description | Data deposited in or computed by PubChem | |
Canonical SMILES |
CCC(C)C(C(CC(=O)N1CCCC1C(C(C)C(=O)NC(CC2=CC=CC=C2)C3=NC=CS3)OC)OC)N(C)C(=O)C(C(C)C)NC(=O)C(C(C)C)NC | |
Source | PubChem | |
URL | https://pubchem.ncbi.nlm.nih.gov | |
Description | Data deposited in or computed by PubChem | |
Isomeric SMILES |
CC[C@H](C)[C@@H]([C@@H](CC(=O)N1CCC[C@H]1[C@@H]([C@@H](C)C(=O)N[C@@H](CC2=CC=CC=C2)C3=NC=CS3)OC)OC)N(C)C(=O)[C@H](C(C)C)NC(=O)[C@H](C(C)C)NC | |
Source | PubChem | |
URL | https://pubchem.ncbi.nlm.nih.gov | |
Description | Data deposited in or computed by PubChem | |
Molecular Formula |
C41H66N6O6S | |
Source | PubChem | |
URL | https://pubchem.ncbi.nlm.nih.gov | |
Description | Data deposited in or computed by PubChem | |
DSSTOX Substance ID |
DTXSID101010182 | |
Record name | Monomethylauristatin D | |
Source | EPA DSSTox | |
URL | https://comptox.epa.gov/dashboard/DTXSID101010182 | |
Description | DSSTox provides a high quality public chemistry resource for supporting improved predictive toxicology. | |
Molecular Weight |
771.1 g/mol | |
Source | PubChem | |
URL | https://pubchem.ncbi.nlm.nih.gov | |
Description | Data deposited in or computed by PubChem | |
CAS No. |
203849-91-6 | |
Record name | Monomethylauristatin D | |
Source | EPA DSSTox | |
URL | https://comptox.epa.gov/dashboard/DTXSID101010182 | |
Description | DSSTox provides a high quality public chemistry resource for supporting improved predictive toxicology. | |
Retrosynthesis Analysis
AI-Powered Synthesis Planning: Our tool employs the Template_relevance Pistachio, Template_relevance Bkms_metabolic, Template_relevance Pistachio_ringbreaker, Template_relevance Reaxys, Template_relevance Reaxys_biocatalysis model, leveraging a vast database of chemical reactions to predict feasible synthetic routes.
One-Step Synthesis Focus: Specifically designed for one-step synthesis, it provides concise and direct routes for your target compounds, streamlining the synthesis process.
Accurate Predictions: Utilizing the extensive PISTACHIO, BKMS_METABOLIC, PISTACHIO_RINGBREAKER, REAXYS, REAXYS_BIOCATALYSIS database, our tool offers high-accuracy predictions, reflecting the latest in chemical research and data.
Strategy Settings
Precursor scoring | Relevance Heuristic |
---|---|
Min. plausibility | 0.01 |
Model | Template_relevance |
Template Set | Pistachio/Bkms_metabolic/Pistachio_ringbreaker/Reaxys/Reaxys_biocatalysis |
Top-N result to add to graph | 6 |
Feasible Synthetic Routes
Q1: What is MMAD and why is it important in aerosol science?
A1: this compound stands for mass median aerodynamic diameter. It is a crucial parameter for characterizing the size distribution of particles in an aerosol. This compound represents the aerodynamic diameter where 50% of the aerosol's mass is contained in particles smaller than that diameter, and 50% is contained in particles larger. Understanding this compound is critical for predicting aerosol behavior, including deposition patterns in the respiratory system, environmental transport, and efficacy of inhaled medications.
Q2: How does particle size, specifically this compound, influence the deposition of inhaled aerosols in the respiratory tract?
A2: Particle size, as represented by this compound, significantly impacts the deposition of inhaled aerosols in the respiratory tract. Larger particles (this compound > 5 μm) tend to deposit in the oropharyngeal region due to inertial impaction. [, ] Particles in the 2-5 μm range deposit primarily in the central airways (trachea and bronchi) by sedimentation. [, ] Smaller particles (< 2 μm) can reach the deep lung, depositing in the bronchioles and alveoli via Brownian diffusion. [, ] Optimizing this compound is crucial for ensuring targeted drug delivery to specific regions of the lungs. []
Q3: What methods are commonly used to measure the size distribution and this compound of aerosols?
A3: Several methods can determine the size distribution and this compound of aerosols. Cascade impaction is a widely used technique that separates particles based on their aerodynamic diameter as they impact on different stages of the impactor. [, , ] Other methods include time-of-flight analysis using instruments like the Aerodynamic Particle Sizer Spectrometer (APS) [, ] and laser diffraction techniques. [] The choice of method depends on factors such as the aerosol properties, required measurement range, and desired accuracy.
Q4: How does relative humidity affect the this compound of hygroscopic aerosols?
A4: Hygroscopic aerosols, like those produced by some inhalers, grow in size with increasing humidity due to water vapor condensation on the particles. [, ] This growth can substantially increase the this compound, affecting the aerosol's deposition pattern in the respiratory tract. [] For instance, the this compound of cromolyn sodium powder dispersed by a Spinhaler® significantly increased with rising humidity. []
Q5: How does the use of a spacer device impact the this compound and deposition of aerosols from pressurized metered-dose inhalers (pMDIs)?
A5: Spacer devices attached to pMDIs can improve drug delivery to the lungs by modifying the aerosol characteristics. They allow the propellant to evaporate before inhalation, reducing initial droplet size and this compound. [] Spacers also decrease the velocity of the aerosol, minimizing oropharyngeal deposition and increasing the fraction of drug reaching the lower airways. [] Studies on betamethasone valerate and triamcinolone acetonide pMDI formulations demonstrated that incorporating a spacer device effectively reduced this compound to less than 4.7 μm and increased the fine particle fraction (FPF). []
Q6: Can the choice of nebulizer and compressor combination influence the this compound and delivery efficiency of nebulized medications?
A6: Yes, the choice of nebulizer and compressor combination significantly influences the this compound and delivery efficiency of nebulized medications. Different nebulizer designs and operating pressures can produce aerosols with varying particle size distributions and output volumes. [] The pressure-flow characteristics of the compressor also impact the nebulizer's performance. [] Using mismatched nebulizers and compressors can lead to suboptimal drug delivery and treatment outcomes. []
Q7: Can you provide an example of a dry-powder formulation for inhalation and its characteristics related to this compound?
A7: A dry-powder formulation of nanocrystalline camptothecin (CPT) was developed for inhalation delivery as a potential lung cancer treatment. [] CPT nanocrystals, with an approximate diameter of 120 nm, were spray-dried with dextran, creating particles suitable for lung deposition. [] This formulation achieved an this compound of 2.7 μm and a fine particle fraction (FPF) of 78%, indicating efficient delivery to the deep lung. []
Q8: How can computational chemistry and modeling be applied in understanding and predicting this compound?
A8: Computational chemistry and modeling can help understand the factors influencing this compound and predict the behavior of aerosol particles. Molecular dynamics simulations can investigate the interactions between drug particles, excipients, and solvents during aerosol generation. [] Computational fluid dynamics (CFD) models can simulate aerosol flow and deposition patterns in the respiratory tract, taking into account particle size distribution and breathing parameters. [] These tools facilitate the design and optimization of inhalation formulations with desired this compound characteristics for targeted drug delivery. []
Descargo de responsabilidad e información sobre productos de investigación in vitro
Tenga en cuenta que todos los artículos e información de productos presentados en BenchChem están destinados únicamente con fines informativos. Los productos disponibles para la compra en BenchChem están diseñados específicamente para estudios in vitro, que se realizan fuera de organismos vivos. Los estudios in vitro, derivados del término latino "in vidrio", involucran experimentos realizados en entornos de laboratorio controlados utilizando células o tejidos. Es importante tener en cuenta que estos productos no se clasifican como medicamentos y no han recibido la aprobación de la FDA para la prevención, tratamiento o cura de ninguna condición médica, dolencia o enfermedad. Debemos enfatizar que cualquier forma de introducción corporal de estos productos en humanos o animales está estrictamente prohibida por ley. Es esencial adherirse a estas pautas para garantizar el cumplimiento de los estándares legales y éticos en la investigación y experimentación.