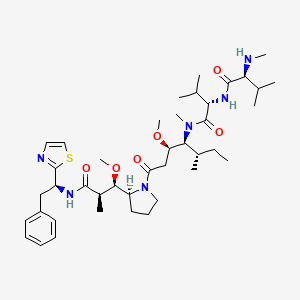
MMAD
Übersicht
Beschreibung
Demethyldolastatin 10, auch bekannt als Monomethyl-Dolastatin 10, ist ein potenter Tubulin-Inhibitor, der aus dem marinen Naturprodukt Dolastatin 10 gewonnen wird. Es ist ein synthetisches Analogon von Dolastatin 10, das ursprünglich aus der indischen Ozean-Schnecke Dolabella auricularia isoliert wurde. Demethyldolastatin 10 hat eine signifikante Antitumoraktivität gezeigt und wird als Toxin-Nutzlast in Antikörper-Wirkstoff-Konjugaten für die gezielte Krebstherapie eingesetzt .
Wissenschaftliche Forschungsanwendungen
Demethyldolastatin 10 hat eine breite Palette von Anwendungen in der wissenschaftlichen Forschung, darunter:
Chemie: Wird als Baustein bei der Synthese komplexer Moleküle und als Reagenz bei verschiedenen chemischen Reaktionen verwendet.
Biologie: Wird für seine Auswirkungen auf zelluläre Prozesse untersucht, insbesondere seine Fähigkeit, die Tubulinpolymerisation zu hemmen.
Medizin: Wird bei der Entwicklung von Antikörper-Wirkstoff-Konjugaten für die gezielte Krebstherapie eingesetzt. .
Industrie: Wird bei der Herstellung von Antikörper-Wirkstoff-Konjugaten für pharmazeutische Anwendungen eingesetzt
Wirkmechanismus
Demethyldolastatin 10 übt seine Wirkung aus, indem es die Tubulinpolymerisation hemmt, was die Bildung von Mikrotubuli stört. Diese Hemmung führt zu einem Zellzyklusarrest und Apoptose in Krebszellen. Die molekularen Ziele von Demethyldolastatin 10 umfassen Tubulin, und es beeinflusst Signalwege, die an Zellteilung und Apoptose beteiligt sind .
Wirkmechanismus
Target of Action
Monomethyl Auristatin E (MMAE), also known as Mmad, primarily targets tubulin , a globular protein that is the main constituent of microtubules in cells . Tubulin plays a crucial role in maintaining cell structure, chromosome segregation, and intracellular transport .
Mode of Action
MMAE is an antimitotic agent that inhibits cell division by blocking the polymerization of tubulin . It is linked to a monoclonal antibody (MAB) which directs it to the cancer cells . The linker to the monoclonal antibody is stable in extracellular fluid, but is cleaved by cathepsin once the conjugate has entered a tumor cell, thus activating the antimitotic mechanism .
Biochemical Pathways
The primary biochemical pathway affected by MMAE is the microtubule assembly process . By inhibiting tubulin polymerization, MMAE disrupts the formation of the mitotic spindle, which is essential for cell division . This disruption leads to cell cycle arrest at the G2/M phase and ultimately induces apoptosis, or programmed cell death .
Pharmacokinetics
The pharmacokinetics of MMAE involves its absorption, distribution, metabolism, and excretion (ADME) properties. As a component of antibody-drug conjugates (ADCs), MMAE exhibits good pharmacokinetic properties . The linker to the monoclonal antibody is stable in extracellular fluid, but is cleaved by cathepsin once the conjugate has entered a tumor cell, thus releasing MMAE . The released MMAE then exerts its cytotoxic effects .
Result of Action
The result of MMAE’s action is the inhibition of cell division and the induction of apoptosis in cancer cells . By disrupting microtubule dynamics, MMAE prevents cancer cells from properly segregating their chromosomes during mitosis . This disruption leads to cell cycle arrest and ultimately to cell death .
Action Environment
The action of MMAE can be influenced by various environmental factors. For instance, the pH of the tumor microenvironment can affect the stability of the linker that attaches MMAE to the monoclonal antibody . Additionally, the expression levels of the target antigen and the presence of multidrug resistance proteins can also influence the efficacy of MMAE .
Vorbereitungsmethoden
Synthesewege und Reaktionsbedingungen
Die Synthese von Demethyldolastatin 10 umfasst mehrere Schritte, darunter die Kupplung verschiedener Aminosäurereste. Ein Schlüsselschritt ist das stabile Oximglicierungsverfahren, das nahezu homogene Antikörper-Wirkstoff-Konjugate mit einem Arzneimittel-Antikörper-Verhältnis von etwa 2,0 ergibt . Die Löslichkeit dieser Verbindung in Dimethylsulfoxid beträgt über 10 Millimol. Um eine höhere Konzentration zu erhalten, kann die Verbindung 10 Minuten lang bei 37 °C erwärmt und/oder in einem Ultraschallbad geschüttelt werden .
Industrielle Produktionsverfahren
Die industrielle Produktion von Demethyldolastatin 10 beinhaltet in der Regel die großtechnische Synthese unter Verwendung der gleichen Synthesewege wie oben beschrieben. Der Prozess gewährleistet hohe Reinheit und Konsistenz, was für die Verwendung in Antikörper-Wirkstoff-Konjugaten unerlässlich ist .
Analyse Chemischer Reaktionen
Arten von Reaktionen
Demethyldolastatin 10 unterliegt verschiedenen chemischen Reaktionen, darunter:
Oxidation: Diese Reaktion beinhaltet die Addition von Sauerstoff oder die Entfernung von Wasserstoff.
Reduktion: Diese Reaktion beinhaltet die Addition von Wasserstoff oder die Entfernung von Sauerstoff.
Substitution: Diese Reaktion beinhaltet die Ersetzung eines Atoms oder einer Atomgruppe durch ein anderes.
Gängige Reagenzien und Bedingungen
Häufig verwendete Reagenzien bei diesen Reaktionen sind Oxidationsmittel wie Kaliumpermanganat, Reduktionsmittel wie Natriumborhydrid und verschiedene Nucleophile für Substitutionsreaktionen. Die Bedingungen für diese Reaktionen variieren je nach gewünschtem Produkt und der durchgeführten Reaktion .
Hauptsächlich gebildete Produkte
Die Hauptprodukte, die aus diesen Reaktionen gebildet werden, umfassen verschiedene Derivate von Demethyldolastatin 10, die für weitere chemische Modifikationen oder als Zwischenprodukte bei der Synthese komplexerer Moleküle verwendet werden können .
Vergleich Mit ähnlichen Verbindungen
Demethyldolastatin 10 ähnelt anderen Tubulin-Inhibitoren, wie zum Beispiel:
Dolastatin 10: Die Stammverbindung, von der Demethyldolastatin 10 abgeleitet ist. .
Monomethyl-Auristatin E: Ein weiteres synthetisches Analogon von Dolastatin 10, das in Antikörper-Wirkstoff-Konjugaten zur Krebstherapie eingesetzt wird
Vinblastin: Ein Naturprodukt, das die Tubulinpolymerisation hemmt und in der Chemotherapie eingesetzt wird.
Die Einzigartigkeit von Demethyldolastatin 10 liegt in seinen spezifischen Modifikationen, die seine Stabilität und Wirksamkeit in Antikörper-Wirkstoff-Konjugaten verbessern, was es zu einem wertvollen Werkzeug in der gezielten Krebstherapie macht .
Eigenschaften
IUPAC Name |
(2S)-N-[(2S)-1-[[(3R,4S,5S)-3-methoxy-1-[(2S)-2-[(1R,2R)-1-methoxy-2-methyl-3-oxo-3-[[(1S)-2-phenyl-1-(1,3-thiazol-2-yl)ethyl]amino]propyl]pyrrolidin-1-yl]-5-methyl-1-oxoheptan-4-yl]-methylamino]-3-methyl-1-oxobutan-2-yl]-3-methyl-2-(methylamino)butanamide | |
---|---|---|
Source | PubChem | |
URL | https://pubchem.ncbi.nlm.nih.gov | |
Description | Data deposited in or computed by PubChem | |
InChI |
InChI=1S/C41H66N6O6S/c1-12-27(6)36(46(9)41(51)35(26(4)5)45-39(50)34(42-8)25(2)3)32(52-10)24-33(48)47-21-16-19-31(47)37(53-11)28(7)38(49)44-30(40-43-20-22-54-40)23-29-17-14-13-15-18-29/h13-15,17-18,20,22,25-28,30-32,34-37,42H,12,16,19,21,23-24H2,1-11H3,(H,44,49)(H,45,50)/t27-,28+,30-,31-,32+,34-,35-,36-,37+/m0/s1 | |
Source | PubChem | |
URL | https://pubchem.ncbi.nlm.nih.gov | |
Description | Data deposited in or computed by PubChem | |
InChI Key |
BLUGYPPOFIHFJS-UUFHNPECSA-N | |
Source | PubChem | |
URL | https://pubchem.ncbi.nlm.nih.gov | |
Description | Data deposited in or computed by PubChem | |
Canonical SMILES |
CCC(C)C(C(CC(=O)N1CCCC1C(C(C)C(=O)NC(CC2=CC=CC=C2)C3=NC=CS3)OC)OC)N(C)C(=O)C(C(C)C)NC(=O)C(C(C)C)NC | |
Source | PubChem | |
URL | https://pubchem.ncbi.nlm.nih.gov | |
Description | Data deposited in or computed by PubChem | |
Isomeric SMILES |
CC[C@H](C)[C@@H]([C@@H](CC(=O)N1CCC[C@H]1[C@@H]([C@@H](C)C(=O)N[C@@H](CC2=CC=CC=C2)C3=NC=CS3)OC)OC)N(C)C(=O)[C@H](C(C)C)NC(=O)[C@H](C(C)C)NC | |
Source | PubChem | |
URL | https://pubchem.ncbi.nlm.nih.gov | |
Description | Data deposited in or computed by PubChem | |
Molecular Formula |
C41H66N6O6S | |
Source | PubChem | |
URL | https://pubchem.ncbi.nlm.nih.gov | |
Description | Data deposited in or computed by PubChem | |
DSSTOX Substance ID |
DTXSID101010182 | |
Record name | Monomethylauristatin D | |
Source | EPA DSSTox | |
URL | https://comptox.epa.gov/dashboard/DTXSID101010182 | |
Description | DSSTox provides a high quality public chemistry resource for supporting improved predictive toxicology. | |
Molecular Weight |
771.1 g/mol | |
Source | PubChem | |
URL | https://pubchem.ncbi.nlm.nih.gov | |
Description | Data deposited in or computed by PubChem | |
CAS No. |
203849-91-6 | |
Record name | Monomethylauristatin D | |
Source | EPA DSSTox | |
URL | https://comptox.epa.gov/dashboard/DTXSID101010182 | |
Description | DSSTox provides a high quality public chemistry resource for supporting improved predictive toxicology. | |
Retrosynthesis Analysis
AI-Powered Synthesis Planning: Our tool employs the Template_relevance Pistachio, Template_relevance Bkms_metabolic, Template_relevance Pistachio_ringbreaker, Template_relevance Reaxys, Template_relevance Reaxys_biocatalysis model, leveraging a vast database of chemical reactions to predict feasible synthetic routes.
One-Step Synthesis Focus: Specifically designed for one-step synthesis, it provides concise and direct routes for your target compounds, streamlining the synthesis process.
Accurate Predictions: Utilizing the extensive PISTACHIO, BKMS_METABOLIC, PISTACHIO_RINGBREAKER, REAXYS, REAXYS_BIOCATALYSIS database, our tool offers high-accuracy predictions, reflecting the latest in chemical research and data.
Strategy Settings
Precursor scoring | Relevance Heuristic |
---|---|
Min. plausibility | 0.01 |
Model | Template_relevance |
Template Set | Pistachio/Bkms_metabolic/Pistachio_ringbreaker/Reaxys/Reaxys_biocatalysis |
Top-N result to add to graph | 6 |
Feasible Synthetic Routes
Q1: What is MMAD and why is it important in aerosol science?
A1: this compound stands for mass median aerodynamic diameter. It is a crucial parameter for characterizing the size distribution of particles in an aerosol. This compound represents the aerodynamic diameter where 50% of the aerosol's mass is contained in particles smaller than that diameter, and 50% is contained in particles larger. Understanding this compound is critical for predicting aerosol behavior, including deposition patterns in the respiratory system, environmental transport, and efficacy of inhaled medications.
Q2: How does particle size, specifically this compound, influence the deposition of inhaled aerosols in the respiratory tract?
A2: Particle size, as represented by this compound, significantly impacts the deposition of inhaled aerosols in the respiratory tract. Larger particles (this compound > 5 μm) tend to deposit in the oropharyngeal region due to inertial impaction. [, ] Particles in the 2-5 μm range deposit primarily in the central airways (trachea and bronchi) by sedimentation. [, ] Smaller particles (< 2 μm) can reach the deep lung, depositing in the bronchioles and alveoli via Brownian diffusion. [, ] Optimizing this compound is crucial for ensuring targeted drug delivery to specific regions of the lungs. []
Q3: What methods are commonly used to measure the size distribution and this compound of aerosols?
A3: Several methods can determine the size distribution and this compound of aerosols. Cascade impaction is a widely used technique that separates particles based on their aerodynamic diameter as they impact on different stages of the impactor. [, , ] Other methods include time-of-flight analysis using instruments like the Aerodynamic Particle Sizer Spectrometer (APS) [, ] and laser diffraction techniques. [] The choice of method depends on factors such as the aerosol properties, required measurement range, and desired accuracy.
Q4: How does relative humidity affect the this compound of hygroscopic aerosols?
A4: Hygroscopic aerosols, like those produced by some inhalers, grow in size with increasing humidity due to water vapor condensation on the particles. [, ] This growth can substantially increase the this compound, affecting the aerosol's deposition pattern in the respiratory tract. [] For instance, the this compound of cromolyn sodium powder dispersed by a Spinhaler® significantly increased with rising humidity. []
Q5: How does the use of a spacer device impact the this compound and deposition of aerosols from pressurized metered-dose inhalers (pMDIs)?
A5: Spacer devices attached to pMDIs can improve drug delivery to the lungs by modifying the aerosol characteristics. They allow the propellant to evaporate before inhalation, reducing initial droplet size and this compound. [] Spacers also decrease the velocity of the aerosol, minimizing oropharyngeal deposition and increasing the fraction of drug reaching the lower airways. [] Studies on betamethasone valerate and triamcinolone acetonide pMDI formulations demonstrated that incorporating a spacer device effectively reduced this compound to less than 4.7 μm and increased the fine particle fraction (FPF). []
Q6: Can the choice of nebulizer and compressor combination influence the this compound and delivery efficiency of nebulized medications?
A6: Yes, the choice of nebulizer and compressor combination significantly influences the this compound and delivery efficiency of nebulized medications. Different nebulizer designs and operating pressures can produce aerosols with varying particle size distributions and output volumes. [] The pressure-flow characteristics of the compressor also impact the nebulizer's performance. [] Using mismatched nebulizers and compressors can lead to suboptimal drug delivery and treatment outcomes. []
Q7: Can you provide an example of a dry-powder formulation for inhalation and its characteristics related to this compound?
A7: A dry-powder formulation of nanocrystalline camptothecin (CPT) was developed for inhalation delivery as a potential lung cancer treatment. [] CPT nanocrystals, with an approximate diameter of 120 nm, were spray-dried with dextran, creating particles suitable for lung deposition. [] This formulation achieved an this compound of 2.7 μm and a fine particle fraction (FPF) of 78%, indicating efficient delivery to the deep lung. []
Q8: How can computational chemistry and modeling be applied in understanding and predicting this compound?
A8: Computational chemistry and modeling can help understand the factors influencing this compound and predict the behavior of aerosol particles. Molecular dynamics simulations can investigate the interactions between drug particles, excipients, and solvents during aerosol generation. [] Computational fluid dynamics (CFD) models can simulate aerosol flow and deposition patterns in the respiratory tract, taking into account particle size distribution and breathing parameters. [] These tools facilitate the design and optimization of inhalation formulations with desired this compound characteristics for targeted drug delivery. []
Haftungsausschluss und Informationen zu In-Vitro-Forschungsprodukten
Bitte beachten Sie, dass alle Artikel und Produktinformationen, die auf BenchChem präsentiert werden, ausschließlich zu Informationszwecken bestimmt sind. Die auf BenchChem zum Kauf angebotenen Produkte sind speziell für In-vitro-Studien konzipiert, die außerhalb lebender Organismen durchgeführt werden. In-vitro-Studien, abgeleitet von dem lateinischen Begriff "in Glas", beinhalten Experimente, die in kontrollierten Laborumgebungen unter Verwendung von Zellen oder Geweben durchgeführt werden. Es ist wichtig zu beachten, dass diese Produkte nicht als Arzneimittel oder Medikamente eingestuft sind und keine Zulassung der FDA für die Vorbeugung, Behandlung oder Heilung von medizinischen Zuständen, Beschwerden oder Krankheiten erhalten haben. Wir müssen betonen, dass jede Form der körperlichen Einführung dieser Produkte in Menschen oder Tiere gesetzlich strikt untersagt ist. Es ist unerlässlich, sich an diese Richtlinien zu halten, um die Einhaltung rechtlicher und ethischer Standards in Forschung und Experiment zu gewährleisten.