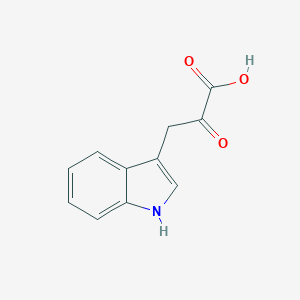
Indole-3-pyruvic acid
Descripción general
Descripción
Indole-3-pyruvic acid (I3PA) is a critical intermediate in the biosynthesis of the phytohormone indole-3-acetic acid (IAA), the primary auxin in plants. Derived from tryptophan via aminotransferase enzymes, I3PA serves as a substrate for YUCCA-family flavin monooxygenases, which catalyze its conversion to IAA . This pathway is evolutionarily conserved across plants, fungi (e.g., Neurospora crassa), and bacteria (e.g., Arthrobacter pascens), underscoring its biological significance in growth and development .
I3PA is chemically unstable and spontaneously degrades into indole-3-lactic acid (ILA) under physiological conditions . Its role extends beyond auxin synthesis: I3PA modulates neurological processes, exhibits antioxidant properties, and serves as a biomarker in Alzheimer’s disease (AD) due to its altered levels in AD patients .
Métodos De Preparación
a. Rutas sintéticas:
El ácido indol-3-pirúvico puede sintetizarse mediante diversos métodos, que incluyen:
Condensación de Knoevenagel: Reaccionar indol-3-acetaldehído con ácido pirúvico o sus derivados .
Conversión enzimática: Utilizar enzimas como la transaminasa del triptófano para convertir el triptófano en ácido indol-3-pirúvico .
b. Producción industrial:
Si bien los métodos de producción a escala industrial son menos comunes, la investigación continúa explorando enfoques eficientes y escalables.
Análisis De Reacciones Químicas
Core Biosynthetic Pathway
IPyA is synthesized through a two-step enzymatic process in the primary auxin biosynthesis pathway:
Step 1: Tryptophan to IPyA
-
Reaction : L-tryptophan undergoes oxidative transamination via pyridoxal 5′-phosphate (PLP)-dependent enzymes (TAA1/TARs), producing IPyA and glutamate .
-
Km Values : The Michaelis constant () for tryptophan in the forward reaction is 43.6 µM , indicating moderate substrate affinity .
Step 2: IPyA to Indole-3-Acetic Acid (IAA)
-
Reaction : IPyA is converted to IAA by YUCCA (YUC) flavin monooxygenases .
-
Alternative Route : In some microbes, IPyA is first decarboxylated to indole-3-acetaldehyde (IAAld) by pyruvate decarboxylase (requiring thiamine pyrophosphate, TPP), followed by oxidation to IAA .
Key Enzymatic Features
Regulatory Mechanisms
Negative Feedback by IPyA
-
IPyA inhibits TAA1/TARs activity via feedback regulation, modulating auxin biosynthesis flux .
-
Equilibrium Shift : Elevated IPyA levels reverse TAA1 activity, converting IPyA back to Trp () when YUC activity is limited .
Chemical Inhibition Studies
-
TAA1/TARs Inhibitors (e.g., KOK2099): Reduce IPyA and IAA levels, confirming pathway dependency .
-
YUC Inhibitors : Block IAA production, validating the two-step mechanism .
Analytical Findings
HPLC Quantification
-
Δcfp Knockout Strain : Produced 64.4% more indole-3-lactic acid (ILA) (15.78 ng) than wild-type (5.62 ng), suggesting metabolic rerouting in IPyA pathways .
-
Δcbs-3;Δahd-2 Double Knockout : Showed a 67% reduction in IAA , highlighting synergistic enzyme roles .
Structural and Mechanistic Insights
-
TPP Binding : Pyruvate decarboxylase’s active site conserves critical residues (e.g., Asp27, His114) for TPP coordination, confirmed by structural alignment (PDB ID: 1OVM) .
-
Substrate Channeling : IPyA’s instability necessitates tight coupling between TAA1 and YUC enzymes to prevent accumulation .
IPyA’s chemical reactions are tightly regulated through enzyme kinetics, feedback mechanisms, and substrate channeling, ensuring precise control over auxin biosynthesis. These insights derive from structural studies, genetic knockouts, and inhibitor experiments, underscoring IPyA’s pivotal role in plant physiology .
Aplicaciones Científicas De Investigación
Role in Plant Development
Auxin Synthesis Pathway
IPyA is a precursor in the biosynthesis of indole-3-acetic acid (IAA), a crucial plant hormone that regulates growth and development. The pathway involves the conversion of tryptophan to IPyA, which is then transformed into IAA. Research indicates that this pathway is particularly active in meristematic regions of plants, such as the liverwort Marchantia polymorpha, where it regulates development and dormancy .
Table 1: Summary of IPyA's Role in Plant Development
Plant Species | Function of IPyA | Reference |
---|---|---|
Marchantia polymorpha | Regulates development and dormancy | |
Various Angiosperms | Precursor for IAA synthesis |
Medical Applications
Rheumatoid Arthritis Treatment
Recent studies have highlighted the potential of IPyA in alleviating rheumatoid arthritis (RA). It acts as a protective factor by restoring the balance between Th17 and Treg cells through the aryl hydrocarbon receptor (AhR) pathway. This balance is critical for managing autoimmune responses .
Case Study: Clinical Evaluation of IPyA in RA
In a controlled study involving rats with collagen-induced arthritis, treatment with IPyA demonstrated significant improvement in clinical scores compared to control groups. The study established that IPyA administration could effectively reduce inflammation and joint damage associated with RA .
Table 2: Effects of IPyA on Rheumatoid Arthritis
Treatment Group | Dosage | Clinical Outcome | Reference |
---|---|---|---|
Control | N/A | No improvement | |
Methotrexate | 1.5 mg/kg | Moderate improvement | |
IPyA-10 | 10 mg/kg | Significant improvement | |
IPyA-20 | 20 mg/kg | Major reduction in symptoms |
Agricultural Applications
Plant Growth Promotion
IPyA has been identified as a key compound in promoting plant growth through its role as a signaling molecule. It enhances root development and nutrient uptake, making it valuable for agricultural practices aimed at improving crop yields.
Biofertilizer Potential
Research on Pantoea agglomerans, a bacterium that produces IPyA, suggests its application as a biofertilizer. This bacterium not only promotes plant growth but also provides resistance against certain pathogens, enhancing overall plant health .
Table 3: Applications of IPyA in Agriculture
Application | Mechanism | Reference |
---|---|---|
Plant Growth Promotion | Enhances root development | |
Biofertilizer | Increases nutrient availability |
Metabolic Studies
Predictive Biomarker for Obesity Treatment
Recent findings suggest that preoperative levels of IPyA may predict fat-free mass changes following sleeve gastrectomy in obese patients. This indicates its potential use as a biomarker for assessing metabolic responses post-surgery .
Mecanismo De Acción
El ácido indol-3-pirúvico activa la vía del AHR, modulando la expresión génica. Sus efectos implican interacciones con elementos sensibles al AHR en genes diana .
Comparación Con Compuestos Similares
Comparison with Structurally and Functionally Related Compounds
The following compounds share structural similarities with I3PA but differ in biosynthesis, stability, and biological roles.
Indole-3-Acetic Acid (IAA)
- Structure : Features an acetic acid side chain instead of I3PA’s α-keto group.
- Biosynthesis : Directly synthesized from I3PA via YUCCA enzymes in the primary auxin pathway .
- Function : Active auxin regulating cell elongation, root development, and embryogenesis. Chemical inhibition of the I3PA pathway reduces somatic embryo formation in plants, highlighting IAA’s dependency on I3PA .
- Regulatory Specificity : In Pseudomonas fluorescens, the transcriptional regulator AdmX binds both I3PA and IAA but only IAA activates antibiotic production, emphasizing its unique signaling role .
Indole-3-Propionic Acid (IPA)
- Structure : Propionic acid side chain replaces I3PA’s α-keto group.
- Biosynthesis : Produced via microbial metabolism of tryptophan or reduction of I3PA derivatives .
- Function: Neuroprotective metabolite with antioxidant activity. Unlike I3PA, IPA is a potent inhibitor of kynurenine aminotransferase-I (KAT-I; IC₅₀ = 0.14 ± 0.20 mM), making it a candidate for treating neurological disorders .
- Gut Microbiota : Elevated in sheep fed high-protein diets, suggesting a role in gut health .
Indole-3-Lactic Acid (ILA)
- Structure : Lactic acid side chain derived from I3PA degradation.
- Biosynthesis : Formed spontaneously from unstable I3PA or via enzymatic reduction .
- Function : Common microbial metabolite (e.g., lactic acid bacteria) with strain-specific production . Inhibits KAT-I (IC₅₀ = 0.22 ± 0.22 mM) but less potently than IPA .
Tryptamine
- Structure : Decarboxylated tryptophan derivative lacking the keto group.
- Biosynthesis: Produced via aromatic-L-amino acid decarboxylase activity, bypassing I3PA .
- Function : Precursor for serotonin and melatonin in animals; in plants, it contributes to IAA synthesis via alternative pathways but is less significant than the I3PA route .
Key Comparative Data
Table 1: Enzyme Inhibition Potency (KAT-I)
Compound | IC₅₀ (mM) | Reference |
---|---|---|
Indole-3-propionic acid | 0.14 ± 0.20 | |
Indole-3-lactic acid | 0.22 ± 0.22 | |
Tryptophan | Less potent | |
Indole-3-pyruvic acid | Less potent |
Table 2: Metabolic Stability and Derivatives
Actividad Biológica
Indole-3-pyruvic acid (IPyA) is a significant metabolite derived from the amino acid tryptophan (Trp) and plays a crucial role in various biological processes, particularly in plant development and stress responses. This compound is integral to the biosynthesis of auxins, which are vital for plant growth and development. Recent studies have highlighted its multifaceted biological activities, including regulatory roles in auxin biosynthesis, immune responses, and potential therapeutic applications in human health.
1. Auxin Biosynthesis Regulation
IPyA is a key intermediate in the auxin biosynthesis pathway, which converts Trp to indole-3-acetic acid (IAA), the primary plant hormone regulating growth. The pathway involves two main enzymatic steps:
- Trp Aminotransferases (TAA/TARs) convert Trp into IPyA.
- Flavin Monooxygenases (YUCCAs) further convert IPyA into IAA.
Research indicates that IPyA regulates the activity of TAA1/TARs through a negative feedback mechanism, ensuring balanced auxin levels essential for plant development. For instance, in Arabidopsis thaliana, IPyA maintains low levels to prevent excessive IAA formation, with a reported value of 0.7 µM for IPyA compared to 43.6 µM for Trp, indicating its higher affinity and regulatory role in auxin biosynthesis .
2. Role in Plant Development
IPyA has been shown to influence various developmental processes across different plant species:
- In Marchantia polymorpha, IPyA is involved in gametophyte development and dormancy regulation, showcasing its essential role in non-vascular plants .
- Studies on Cicer arietinum (chickpea) demonstrate that IPyA enhances nodulation and biomass when used in conjunction with nitrogen-fixing bacteria, highlighting its importance in symbiotic relationships .
3. Immune Responses and Health Benefits
Recent findings suggest that IPyA may have therapeutic potential in human health:
- It acts as a ligand for the aryl hydrocarbon receptor (AhR), influencing immune responses by promoting the differentiation of T helper cells into regulatory T cells, which are crucial for maintaining immune balance .
- The modulation of immune responses via IPyA could provide insights into treatments for autoimmune diseases such as rheumatoid arthritis .
4. Stress Response Mechanisms
IPyA is implicated in stress response pathways:
- Research indicates that it may play a role in alleviating stress-induced effects in plants by modulating hormonal responses and enhancing resilience against environmental challenges .
Case Study 1: Auxin Production and Plant Growth
Objective: To evaluate the impact of exogenous IPyA on plant growth parameters.
Methodology:
- Plants were treated with varying concentrations of IPyA.
- Growth metrics such as height, leaf number, and root biomass were measured.
Results:
- Treatment with 1 µM IPyA resulted in a statistically significant increase in root biomass compared to controls (p < 0.05).
Treatment Concentration | Root Biomass (g) | Height (cm) | Leaf Number |
---|---|---|---|
Control | 0.5 | 15 | 5 |
0.5 µM | 0.7 | 18 | 6 |
1 µM | 1.2 | 22 | 8 |
Case Study 2: IPyA and Nodulation Enhancement
Objective: To assess the effect of IPyA on nodulation in chickpeas.
Methodology:
- Chickpea seeds were inoculated with Mesorhizobium sp. and treated with varying doses of IPyA.
Results:
- The combination treatment significantly increased nodule number and biomass compared to controls.
Treatment Group | Nodule Number | Nodule Biomass (g) |
---|---|---|
Control | 5 | 0.2 |
Mesorhizobium Only | 8 | 0.4 |
Mesorhizobium + 1 µM IPyA | 12 | 0.6 |
Q & A
Basic Research Questions
Q. What are the primary biosynthetic pathways of I3P in eukaryotic systems, and how do they differ from microbial pathways?
- I3P is a key intermediate in the indole-3-acetic acid (IAA) biosynthesis pathway. In plants and fungi (e.g., Neurospora crassa), the indole-3-pyruvic acid (IPA) pathway involves enzymatic decarboxylation of tryptophan via tryptophan transaminases to produce I3P, followed by oxidative decarboxylation to IAA . In bacteria (e.g., Enterobacter spp.), the ipdC gene encodes indole-3-pyruvate decarboxylase, which catalyzes I3P conversion to indole-3-acetaldehyde, a precursor to IAA . Key differences include enzyme specificity and regulatory mechanisms across kingdoms.
Q. Which analytical methods are most reliable for quantifying I3P in biological samples?
- High-performance liquid chromatography (HPLC) coupled with mass spectrometry (LC-MS/MS) is the gold standard for detecting I3P due to its sensitivity in separating tautomeric forms (keto-enol equilibrium) . Nuclear magnetic resonance (NMR) spectroscopy, particularly ¹H-¹³C HMBC, is critical for structural elucidation and tracking tautomerization dynamics in solution . For microbial studies, intermediate assays using genetic knockouts (e.g., ipdC mutants) validate pathway specificity .
Q. What role does I3P play in cross-kingdom signaling between microbes and plants?
- I3P-derived IAA from plant growth-promoting bacteria (e.g., Enterobacter spp.) enhances root elongation and nutrient uptake in host plants. Experimental designs often involve co-culturing mutants (e.g., I3P-deficient strains) with plants (e.g., maize) to measure growth parameters (root length, biomass) under controlled conditions .
Advanced Research Questions
Q. How can contradictory data on I3P’s stability and tautomeric equilibrium be resolved in experimental settings?
- I3P’s keto-enol tautomerism complicates its quantification. Researchers must standardize buffer conditions (pH 7.4, 37°C) and use stabilizing agents (e.g., antioxidants) during extraction. Multi-dimensional NMR (e.g., COSY, HMBC) and isotopic labeling (e.g., ¹³C-tracing) can differentiate tautomers and validate degradation products . Discrepancies in pathway efficiency (e.g., fungal vs. bacterial systems) may arise from enzyme kinetics or competing metabolic routes .
Q. What are the implications of I3P-derived aryl hydrocarbon receptor (AhR) agonists in mammalian systems?
- Aerobic incubation of I3P generates diindole compounds (e.g., indolo[3,2-b]carbazole) that act as AhR ligands, modulating immune responses and xenobiotic metabolism. Fractionation via HPLC and AhR reporter assays (e.g., luciferase-based) are used to isolate and characterize bioactive derivatives . This intersects with neurodegenerative research, as elevated fecal I3P in Alzheimer’s patients correlates with gut microbiota dysbiosis .
Q. How can synthetic biology tools optimize I3P pathway engineering for secondary metabolite production?
- In synthetic minimal cells, I3P serves as a precursor for violacein, an antimicrobial pigment. Modular genetic circuits (e.g., violacein pathway genes under inducible promoters) and programmable cell-cell mating enable tunable production. Chromatographic validation (e.g., HPLC with authentic standards) ensures pathway fidelity . Challenges include balancing flux between competing pathways (e.g., IAA vs. violacein) .
Q. Methodological Considerations
Q. What experimental controls are essential for validating I3P’s role in auxin biosynthesis?
- (i) Use pathway-specific inhibitors (e.g., aminooxyacetic acid for transaminase blockade). (ii) Employ genetic knockouts (e.g., Neurospora Δmao-1 mutants) to disrupt I3P-to-IAA conversion . (iii) Quantify intermediates via LC-MS/MS with internal standards (e.g., deuterated I3P) .
Q. How do researchers address I3P’s oxidative instability in long-term studies?
- Store samples at -20°C under inert gas (N₂/Ar) to prevent degradation. Add chelating agents (e.g., EDTA) to inhibit metal-catalyzed oxidation. Real-time stability assays under varying conditions (pH, temperature) provide degradation kinetics .
Q. Data Interpretation and Conflict Resolution
Q. Why do fungal I3P pathways show lower IAA yields compared to bacterial systems?
- Fungal pathways (e.g., Neurospora crassa) may involve feedback inhibition or competing routes (e.g., tryptophan catabolism to kynurenine). Comparative genomics reveals absent homologs of bacterial ipdC, suggesting divergent evolutionary pressures .
Q. How can metabolomic datasets reconcile I3P’s dual roles as a growth promoter and potential toxin?
- Systems biology approaches (e.g., flux balance analysis) model concentration-dependent effects. For example, low I3P enhances plant growth via IAA, while excess I3P generates cytotoxic AhR ligands . Dose-response assays and transcriptomics (e.g., RNA-seq of exposed tissues) clarify threshold effects.
Propiedades
IUPAC Name |
3-(1H-indol-3-yl)-2-oxopropanoic acid | |
---|---|---|
Source | PubChem | |
URL | https://pubchem.ncbi.nlm.nih.gov | |
Description | Data deposited in or computed by PubChem | |
InChI |
InChI=1S/C11H9NO3/c13-10(11(14)15)5-7-6-12-9-4-2-1-3-8(7)9/h1-4,6,12H,5H2,(H,14,15) | |
Source | PubChem | |
URL | https://pubchem.ncbi.nlm.nih.gov | |
Description | Data deposited in or computed by PubChem | |
InChI Key |
RSTKLPZEZYGQPY-UHFFFAOYSA-N | |
Source | PubChem | |
URL | https://pubchem.ncbi.nlm.nih.gov | |
Description | Data deposited in or computed by PubChem | |
Canonical SMILES |
C1=CC=C2C(=C1)C(=CN2)CC(=O)C(=O)O | |
Source | PubChem | |
URL | https://pubchem.ncbi.nlm.nih.gov | |
Description | Data deposited in or computed by PubChem | |
Molecular Formula |
C11H9NO3 | |
Source | PubChem | |
URL | https://pubchem.ncbi.nlm.nih.gov | |
Description | Data deposited in or computed by PubChem | |
DSSTOX Substance ID |
DTXSID3042053 | |
Record name | Indole-3-pyruvic acid | |
Source | EPA DSSTox | |
URL | https://comptox.epa.gov/dashboard/DTXSID3042053 | |
Description | DSSTox provides a high quality public chemistry resource for supporting improved predictive toxicology. | |
Molecular Weight |
203.19 g/mol | |
Source | PubChem | |
URL | https://pubchem.ncbi.nlm.nih.gov | |
Description | Data deposited in or computed by PubChem | |
CAS No. |
392-12-1 | |
Record name | Indole-3-pyruvic acid | |
Source | CAS Common Chemistry | |
URL | https://commonchemistry.cas.org/detail?cas_rn=392-12-1 | |
Description | CAS Common Chemistry is an open community resource for accessing chemical information. Nearly 500,000 chemical substances from CAS REGISTRY cover areas of community interest, including common and frequently regulated chemicals, and those relevant to high school and undergraduate chemistry classes. This chemical information, curated by our expert scientists, is provided in alignment with our mission as a division of the American Chemical Society. | |
Explanation | The data from CAS Common Chemistry is provided under a CC-BY-NC 4.0 license, unless otherwise stated. | |
Record name | Indol-3-yl pyruvic acid | |
Source | ChemIDplus | |
URL | https://pubchem.ncbi.nlm.nih.gov/substance/?source=chemidplus&sourceid=0000392121 | |
Description | ChemIDplus is a free, web search system that provides access to the structure and nomenclature authority files used for the identification of chemical substances cited in National Library of Medicine (NLM) databases, including the TOXNET system. | |
Record name | Indole-3-pyruvic acid | |
Source | DTP/NCI | |
URL | https://dtp.cancer.gov/dtpstandard/servlet/dwindex?searchtype=NSC&outputformat=html&searchlist=88874 | |
Description | The NCI Development Therapeutics Program (DTP) provides services and resources to the academic and private-sector research communities worldwide to facilitate the discovery and development of new cancer therapeutic agents. | |
Explanation | Unless otherwise indicated, all text within NCI products is free of copyright and may be reused without our permission. Credit the National Cancer Institute as the source. | |
Record name | Indole-3-pyruvic acid | |
Source | EPA DSSTox | |
URL | https://comptox.epa.gov/dashboard/DTXSID3042053 | |
Description | DSSTox provides a high quality public chemistry resource for supporting improved predictive toxicology. | |
Record name | Indole-3-pyruvic acid monohydrate | |
Source | European Chemicals Agency (ECHA) | |
URL | https://echa.europa.eu/substance-information/-/substanceinfo/100.006.250 | |
Description | The European Chemicals Agency (ECHA) is an agency of the European Union which is the driving force among regulatory authorities in implementing the EU's groundbreaking chemicals legislation for the benefit of human health and the environment as well as for innovation and competitiveness. | |
Explanation | Use of the information, documents and data from the ECHA website is subject to the terms and conditions of this Legal Notice, and subject to other binding limitations provided for under applicable law, the information, documents and data made available on the ECHA website may be reproduced, distributed and/or used, totally or in part, for non-commercial purposes provided that ECHA is acknowledged as the source: "Source: European Chemicals Agency, http://echa.europa.eu/". Such acknowledgement must be included in each copy of the material. ECHA permits and encourages organisations and individuals to create links to the ECHA website under the following cumulative conditions: Links can only be made to webpages that provide a link to the Legal Notice page. | |
Record name | INDOLE-3-PYRUVIC ACID | |
Source | FDA Global Substance Registration System (GSRS) | |
URL | https://gsrs.ncats.nih.gov/ginas/app/beta/substances/4QM0LT13A8 | |
Description | The FDA Global Substance Registration System (GSRS) enables the efficient and accurate exchange of information on what substances are in regulated products. Instead of relying on names, which vary across regulatory domains, countries, and regions, the GSRS knowledge base makes it possible for substances to be defined by standardized, scientific descriptions. | |
Explanation | Unless otherwise noted, the contents of the FDA website (www.fda.gov), both text and graphics, are not copyrighted. They are in the public domain and may be republished, reprinted and otherwise used freely by anyone without the need to obtain permission from FDA. Credit to the U.S. Food and Drug Administration as the source is appreciated but not required. | |
Record name | Indolepyruvate | |
Source | Human Metabolome Database (HMDB) | |
URL | http://www.hmdb.ca/metabolites/HMDB0060484 | |
Description | The Human Metabolome Database (HMDB) is a freely available electronic database containing detailed information about small molecule metabolites found in the human body. | |
Explanation | HMDB is offered to the public as a freely available resource. Use and re-distribution of the data, in whole or in part, for commercial purposes requires explicit permission of the authors and explicit acknowledgment of the source material (HMDB) and the original publication (see the HMDB citing page). We ask that users who download significant portions of the database cite the HMDB paper in any resulting publications. | |
Synthesis routes and methods I
Procedure details
Synthesis routes and methods II
Procedure details
Synthesis routes and methods III
Procedure details
Retrosynthesis Analysis
AI-Powered Synthesis Planning: Our tool employs the Template_relevance Pistachio, Template_relevance Bkms_metabolic, Template_relevance Pistachio_ringbreaker, Template_relevance Reaxys, Template_relevance Reaxys_biocatalysis model, leveraging a vast database of chemical reactions to predict feasible synthetic routes.
One-Step Synthesis Focus: Specifically designed for one-step synthesis, it provides concise and direct routes for your target compounds, streamlining the synthesis process.
Accurate Predictions: Utilizing the extensive PISTACHIO, BKMS_METABOLIC, PISTACHIO_RINGBREAKER, REAXYS, REAXYS_BIOCATALYSIS database, our tool offers high-accuracy predictions, reflecting the latest in chemical research and data.
Strategy Settings
Precursor scoring | Relevance Heuristic |
---|---|
Min. plausibility | 0.01 |
Model | Template_relevance |
Template Set | Pistachio/Bkms_metabolic/Pistachio_ringbreaker/Reaxys/Reaxys_biocatalysis |
Top-N result to add to graph | 6 |
Feasible Synthetic Routes
Descargo de responsabilidad e información sobre productos de investigación in vitro
Tenga en cuenta que todos los artículos e información de productos presentados en BenchChem están destinados únicamente con fines informativos. Los productos disponibles para la compra en BenchChem están diseñados específicamente para estudios in vitro, que se realizan fuera de organismos vivos. Los estudios in vitro, derivados del término latino "in vidrio", involucran experimentos realizados en entornos de laboratorio controlados utilizando células o tejidos. Es importante tener en cuenta que estos productos no se clasifican como medicamentos y no han recibido la aprobación de la FDA para la prevención, tratamiento o cura de ninguna condición médica, dolencia o enfermedad. Debemos enfatizar que cualquier forma de introducción corporal de estos productos en humanos o animales está estrictamente prohibida por ley. Es esencial adherirse a estas pautas para garantizar el cumplimiento de los estándares legales y éticos en la investigación y experimentación.