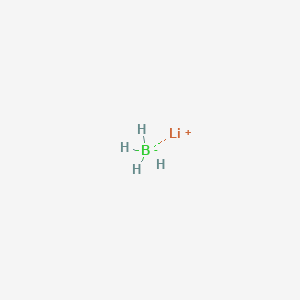
Lithium tetrahydroborate
- 点击 快速询问 获取最新报价。
- 提供有竞争力价格的高质量产品,您可以更专注于研究。
描述
Lithium tetrahydroborate, also known as lithium tetrahydridoborate, is a chemical compound with the formula LiBH₄. It is a white crystalline solid that is highly soluble in ethers and reacts with water. This compound is known for its strong reducing properties and is commonly used in organic synthesis and as a hydrogen storage material.
准备方法
Lithium tetrahydroborate can be synthesized through several methods. One common method involves the metathesis reaction between sodium borohydride and lithium bromide:
NaBH4+LiBr→NaBr+LiBH4
Another method involves the reaction of boron trifluoride with lithium hydride in diethyl ether:
BF3+4LiH→LiBH4+3LiF
These reactions are typically carried out under controlled conditions to ensure the purity and yield of the product.
化学反应分析
Lithium tetrahydroborate is a versatile reducing agent and can participate in various chemical reactions:
Reduction Reactions: It can reduce esters to alcohols, nitriles, and primary amides to amines, and can open epoxides.
Hydrogen Generation: It reacts with water to produce hydrogen gas, which can be used for hydrogen generation.
Reactions with Carbonyl Compounds: This compound can react with a range of carbonyl substrates and other polarized carbon structures to form hydrogen-carbon bonds.
科学研究应用
Lithium tetrahydroborate has a wide range of applications in scientific research:
Biology: Its reducing properties are utilized in various biochemical applications, including the reduction of biomolecules.
Medicine: Research is ongoing into its potential use in drug synthesis and other pharmaceutical applications.
作用机制
The mechanism by which lithium boranuide exerts its effects is primarily through its ability to donate hydride ions (H⁻). This makes it a powerful reducing agent, capable of transferring electrons to other molecules and thereby reducing them. The lithium cation (Li⁺) also plays a role in stabilizing the intermediate complexes formed during these reactions .
相似化合物的比较
Lithium tetrahydroborate can be compared with other similar compounds such as:
Sodium borohydride (NaBH₄): While both are reducing agents, lithium boranuide is stronger and more reactive than sodium borohydride.
Lithium aluminium hydride (LiAlH₄): Lithium aluminium hydride is a more powerful reducing agent than lithium boranuide but is also more hazardous to handle.
Lithium tetrakis(pentafluorophenyl)borate: This compound is used in different applications, primarily in the preparation of cationic transition metal complexes.
This compound stands out due to its balance of reactivity and safety, making it a valuable reagent in both laboratory and industrial settings.
属性
分子式 |
BH4Li |
---|---|
分子量 |
21.8 g/mol |
IUPAC 名称 |
lithium;boranuide |
InChI |
InChI=1S/BH4.Li/h1H4;/q-1;+1 |
InChI 键 |
UUKMSDRCXNLYOO-UHFFFAOYSA-N |
规范 SMILES |
[Li+].[BH4-] |
产品来源 |
United States |
体外研究产品的免责声明和信息
请注意,BenchChem 上展示的所有文章和产品信息仅供信息参考。 BenchChem 上可购买的产品专为体外研究设计,这些研究在生物体外进行。体外研究,源自拉丁语 "in glass",涉及在受控实验室环境中使用细胞或组织进行的实验。重要的是要注意,这些产品没有被归类为药物或药品,他们没有得到 FDA 的批准,用于预防、治疗或治愈任何医疗状况、疾病或疾病。我们必须强调,将这些产品以任何形式引入人类或动物的身体都是法律严格禁止的。遵守这些指南对确保研究和实验的法律和道德标准的符合性至关重要。