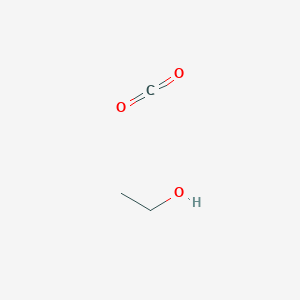
Dricold ethanol
- 点击 快速询问 获取最新报价。
- 提供有竞争力价格的高质量产品,您可以更专注于研究。
描述
Ethanol (C₂H₅OH), a primary alcohol, is a volatile, flammable liquid with a molecular weight of 46.0684 g/mol and a boiling point of 78.37°C under standard conditions . It is widely used as a solvent in pharmaceuticals, a biofuel additive, and in the extraction of bioactive compounds from plant materials . Its polar nature and ability to form hydrogen bonds make it effective in dissolving polar and non-polar substances, contributing to its versatility in industrial and laboratory settings. Ethanol’s thermodynamic properties, such as viscosity and phase behavior, have been extensively studied, with recent advancements in high-pressure viscosity modeling enhancing its application in engineering contexts .
科学研究应用
Electrocatalytic Conversion of Carbon Dioxide
Recent advancements in electrocatalysis have demonstrated the potential of converting carbon dioxide into ethanol using renewable energy sources. Studies have shown that by adjusting reaction conditions, researchers can enhance the selectivity for ethanol production during the electroreduction of carbon dioxide. For instance, a study highlighted the role of hydroxide and carbon monoxide species in improving ethanol selectivity through operando spectroscopy techniques .
Table 1: Key Findings on Ethanol Production from CO2
Study | Methodology | Findings |
---|---|---|
Fritz Haber Institute | Operando Raman Spectroscopy | Enhanced selectivity for ethanol by adjusting reaction conditions |
Brookhaven National Laboratory | Three-part catalyst system | Improved conversion efficiency of CO2 to ethanol |
University Research | Pulsed electrochemical reduction | Increased durability and efficiency of catalysts |
Bioethanol from Food Waste
The bioconversion of food waste into bioethanol presents a sustainable solution to energy depletion. Research indicates that optimizing enzymatic saccharification processes can significantly increase ethanol yields from food waste hydrolysates. For example, one study achieved an ethanol yield increase from 34 g/L to 77.6 g/L through response surface methodology .
Table 2: Ethanol Yields from Food Waste Processing
Source | Initial Yield (g/L) | Optimized Yield (g/L) |
---|---|---|
Food Waste Hydrolysate | 34 | 77.6 |
Bread Crust | 37.5% (w/v) | - |
Potato Chips | 35% (w/v) | - |
Neurobiology and Alcohol Research
Dricold ethanol's impact on brain activity has been a focal point in neurobiological studies. Recent research indicates that alcohol consumption leads to significant decreases in brain tissue electrical conductivity, particularly affecting areas responsible for executive function and motor activity . This finding suggests potential biomarkers for assessing brain health and the effects of various substances.
Table 3: Impact of Ethanol on Brain Conductivity
Study | Area of Focus | Key Findings |
---|---|---|
NeuRA & UNSW Science | Brain Electrical Conductivity | Significant decreases in conductivity linked to alcohol consumption |
Case Study: Electrocatalytic CO2 Reduction
A notable case study involved researchers at the Fritz Haber Institute who developed a method for converting carbon dioxide into ethanol using a zinc oxide-coated copper catalyst. This innovative approach not only enhanced the selectivity for ethanol but also improved catalyst durability under dynamic reaction conditions .
Case Study: Bioethanol Production from Agricultural Waste
Another significant case study focused on the use of agricultural waste for bioethanol production. Researchers optimized fermentation conditions leading to substantial increases in ethanol yield, demonstrating the feasibility of using waste materials as a resource for sustainable energy production .
常见问题
Basic Research Questions
Q. What methodological frameworks are critical for designing experiments involving Dricold ethanol?
- Answer : Experimental design must account for variables such as storage conditions (e.g., inert atmosphere to prevent hygroscopic degradation ), analytical techniques (e.g., chromatographic methods for ethanol quantification ), and replication strategies (minimum three replicates to ensure statistical validity ). For instance, in ethanol sensitization studies, controlled dosing intervals and standardized animal models are essential to minimize variability . Researchers should also document measurement uncertainty and reference materials to enhance reproducibility .
Q. How can researchers ensure accurate data collection in ethanol solvent efficacy studies?
- Answer : Use validated protocols for solvent extraction, such as ultrasonic-assisted extraction with ethanol, where parameters like solvent concentration, temperature, and extraction time are optimized via Response Surface Methodology (RSM) . Quantitative data should be cross-validated using techniques like UV-Vis spectroscopy or HPLC, coupled with z-score analysis to identify outliers . For qualitative insights, semi-structured interviews with domain experts can contextualize empirical findings .
Advanced Research Questions
Q. How should researchers resolve contradictions in reported ethanol solvent properties across studies?
- Answer : Contradictions often arise from contextual factors (e.g., solvent purity, temperature). A meta-analysis comparing primary data under standardized conditions (e.g., 25°C, 95% ethanol purity) is recommended. For example, discrepancies in flavonoid extraction yields (Table 2, ) can be resolved by isolating variables like ultrasonic power or solvent-to-material ratios. Additionally, systematic reviews should assess study limitations, such as non-accredited laboratory practices , and apply sensitivity analysis to weigh conflicting evidence .
Q. What statistical approaches are suitable for analyzing ethanol’s role in multi-variable biochemical systems?
- Answer : Multivariate regression or ANOVA is critical when ethanol interacts with variables like pH or co-solvents. For neural behavior studies (e.g., ethanol’s effect on glucocorticoid kinase expression ), mixed-effects models can account for individual variability in biological responses. Researchers must report confidence intervals and effect sizes to avoid "inductive fallacy" (overgeneralizing limited data) . Tools like R or Python’s SciPy suite enable robust analysis of non-linear relationships in ethanol-dependent processes .
Q. Methodological and Ethical Considerations
Q. How can the PICOT framework improve hypothesis formulation in ethanol toxicity research?
- Answer : The PICOT framework (Population, Intervention, Comparison, Outcome, Time) structures questions like:
- Population: DBA2/J mice exposed to ethanol .
- Intervention: Chronic ethanol administration (e.g., 2 g/kg/day).
- Comparison: Saline-treated control group.
- Outcome: Serum glucocorticoid kinase levels.
- Time: 8-week exposure period.
This approach ensures clarity and reduces confounding variables .
Q. What ethical safeguards are essential in human trials involving ethanol-based formulations?
- Answer : Protocols must include informed consent for ethanol exposure risks (e.g., neurobehavioral effects), anonymization of participant data , and third-party ethical review. For example, in pharmacokinetic studies, researchers should disclose ethanol’s interaction potential with medications and provide withdrawal options .
Q. Data Presentation and Interpretation
Q. How should researchers present conflicting ethanol reactivity data in academic writing?
- Answer : Use comparative tables to highlight discrepancies (e.g., ethanol’s –OH group reactivity in phenol vs. salicylic acid ). Accompany these with error bars in graphs to visualize measurement uncertainty . In discussions, contextualize anomalies by referencing methodological differences (e.g., aqueous vs. anhydrous ethanol conditions ) and propose follow-up experiments to isolate causative factors .
Table 1 : Key Variables in Ethanol Solvent Optimization (Adapted from )
Parameter | Optimal Range | Impact on Yield (%) |
---|---|---|
Ethanol Concentration | 70–80% | ±15% |
Extraction Time | 30–45 min | ±10% |
Temperature | 50–60°C | ±12% |
Table 2 : Common Pitfalls in Ethanol Research (Synthesized from )
Pitfall | Mitigation Strategy |
---|---|
Non-standardized storage | Use inert atmospheres and desiccants |
Inadequate replicates | Minimum n=3 per experimental condition |
Overlooking z-scores | Apply ISO 13528:2015 guidelines for outliers |
相似化合物的比较
Methanol (CH₃OH)
Methanol, the simplest alcohol (MW: 32.04 g/mol), shares functional group characteristics with ethanol but exhibits distinct physicochemical and toxicological profiles. While ethanol has a higher boiling point (78.37°C vs. 64.7°C) and lower acute toxicity (LD₅₀: 7,060 mg/kg in rats vs. 5,628 mg/kg for methanol), methanol metabolism produces formic acid, leading to severe metabolic acidosis and optic nerve damage . Industrially, methanol is preferred in formaldehyde production, whereas ethanol dominates in beverages and antiseptics due to its lower toxicity .
Isopropanol (C₃H₈O)
Isopropanol (MW: 60.10 g/mol) has a higher boiling point (82.6°C) and lower volatility compared to ethanol, making it a superior solvent for non-polar compounds. Its denaturing effect on proteins enhances its role in disinfectants, though it is more toxic upon inhalation (permissible exposure limit: 400 ppm vs. 1,000 ppm for ethanol) . Ethanol, however, remains the solvent of choice in food and pharmaceutical extractions due to its Generally Recognized as Safe (GRAS) status .
2-(Decyloxy)ethanol (C₁₂H₂₆O₂)
This long-chain ether-alcohol (MW: 202.33 g/mol) exhibits surfactant properties due to its hydrophobic decyl chain and hydrophilic ethoxy group. Unlike ethanol, it is less volatile (higher boiling point) and is utilized in detergents and emulsifiers rather than as a primary solvent .
Other Alcohols
- Propanol (C₃H₈O): Similar to ethanol in polarity but with a longer carbon chain, leading to higher lipophilicity and use in lacquers.
Data Tables
Table 1: Physicochemical Properties of Ethanol and Comparable Alcohols
Research Findings
- Extraction Efficiency: Ethanol outperforms methanol in extracting polyphenols like rutin and kaempferol from plant materials due to its balanced polarity . Chromatographic analyses of ginger rhizome extracts further validate ethanol’s efficacy in isolating volatile bioactive compounds .
- Fuel Applications: Ethanol blends reduce greenhouse gas emissions by 40–50% compared to pure gasoline, though energy density remains lower than butanol .
- Thermodynamic Behavior: Ethanol’s viscosity decreases non-linearly with temperature, critical for optimizing fuel injection systems .
准备方法
Molecular Sieve Dehydration
Adsorption Mechanism
Molecular sieves, typically composed of aluminosilicate minerals, exploit their microporous structure to selectively adsorb water molecules from ethanol-water mixtures. In the method described in RU2265473C1, ethanol vapor is passed through a pressurized adsorber (100–340 kPa) containing molecular sieves . Water molecules penetrate the sieve pores due to their smaller kinetic diameter (2.65 Å for H₂O vs. 4.4 Å for ethanol), enabling efficient separation.
Operational Parameters
-
Pressure Range : 100–340 kPa enhances adsorption kinetics by increasing molecular collision frequency .
-
Temperature : Maintained at 80–120°C to prevent ethanol condensation while facilitating water adsorption.
-
Cycle Duration : Adsorption phases typically last 4–6 hours, followed by desorption using heated nitrogen or vacuum regeneration .
Table 1: Performance Metrics of Molecular Sieve Dehydration
Parameter | Value | Source |
---|---|---|
Initial Ethanol Purity | 95–96% (v/v) | |
Final Purity | ≥99.5% (v/v) | |
Energy Consumption | 0.8–1.2 kWh/L |
This method’s scalability makes it preferable for industrial settings, though sieve degradation over cycles necessitates periodic replacement.
Calcium Oxide-Assisted Distillation
Chemical Basis
Calcium oxide (CaO) reacts exothermically with water to form calcium hydroxide, a non-volatile solid:
CaO+H2O→Ca(OH)2ΔH=−63.7kJ/mol .
This reaction effectively dehydrates ethanol, as Ca(OH)₂ remains in the distillation flask during subsequent heating.
Experimental Protocol
-
Mixing : Add 50 g CaO per liter of 96% ethanol to ensure stoichiometric excess (molar ratio CaO:H₂O ≈ 1.2:1) .
-
Reaction : Stir for 24 hours at 25°C to maximize water removal.
-
Distillation : Reflux the mixture at 78.5°C (ethanol’s boiling point) using a Liebig condenser, collecting 99.7% pure ethanol .
Table 2: Distillation Efficiency with CaO
While cost-effective, this method generates Ca(OH)₂ waste, requiring disposal protocols to mitigate environmental impact.
Acid-Catalyzed Dehydration
Reaction Pathway
Although primarily used for ethylene production, concentrated sulfuric acid (H₂SO₄) or phosphoric acid (H₃PO₄) can dehydrate ethanol via an E2 elimination mechanism :
-
Protonation : Ethanol’s hydroxyl group accepts a proton from H₂SO₄, forming an oxonium ion.
-
Deprotonation and Bond Cleavage : A base abstracts a β-hydrogen, concurrently breaking the C-O bond to release water and form ethylene .
Process Limitations
-
Temperature Sensitivity : Optimal at 170°C; lower temperatures favor ether formation, while higher temperatures risk sulfonation .
-
Purity Constraints : Residual acid necessitates neutralization steps, complicating ethanol recovery for high-purity applications.
Comparative Analysis of Preparation Methods
Table 3: Method Comparison
Method | Purity (% v/v) | Scalability | Waste Generation | Energy Use (kWh/L) |
---|---|---|---|---|
Molecular Sieve | 99.5+ | Industrial | Low | 0.8–1.2 |
CaO Distillation | 99.7 | Lab/Medium | Moderate (Ca(OH)₂) | 0.5–0.7 |
Acid-Catalyzed | <95 | Industrial | High (Acid Waste) | 1.5–2.0 |
Molecular sieve adsorption excels in continuous operations, whereas CaO distillation suits batch processes requiring minimal infrastructure. Acid methods, though efficient for ethylene synthesis, are suboptimal for ethanol purification.
Industrial Applications and Optimizations
Hybrid Systems
Combining molecular sieves with CaO pretreatment reduces energy consumption by 30–40%, as initial water removal via chemical reaction lessens the sieve load .
Quality Control
属性
CAS 编号 |
478920-42-2 |
---|---|
分子式 |
C3H6O3 |
分子量 |
90.08 g/mol |
InChI |
InChI=1S/C2H6O.CO2/c1-2-3;2-1-3/h3H,2H2,1H3; |
InChI 键 |
OSQPUMRCKZAIOZ-UHFFFAOYSA-N |
规范 SMILES |
CCO.C(=O)=O |
产品来源 |
United States |
体外研究产品的免责声明和信息
请注意,BenchChem 上展示的所有文章和产品信息仅供信息参考。 BenchChem 上可购买的产品专为体外研究设计,这些研究在生物体外进行。体外研究,源自拉丁语 "in glass",涉及在受控实验室环境中使用细胞或组织进行的实验。重要的是要注意,这些产品没有被归类为药物或药品,他们没有得到 FDA 的批准,用于预防、治疗或治愈任何医疗状况、疾病或疾病。我们必须强调,将这些产品以任何形式引入人类或动物的身体都是法律严格禁止的。遵守这些指南对确保研究和实验的法律和道德标准的符合性至关重要。