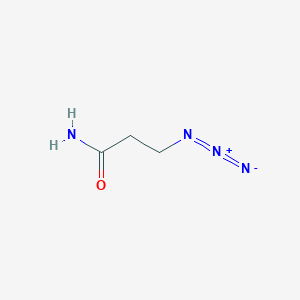
3-Azidopropanamide
- 点击 快速询问 获取最新报价。
- 提供有竞争力价格的高质量产品,您可以更专注于研究。
描述
3-Azidopropanamide is an azide-containing compound characterized by a propionamide backbone with a terminal azido (-N₃) group. These compounds share a three-carbon chain with an azide moiety but differ in their functional groups, influencing their reactivity and applications.
This compound is hypothesized to participate in click chemistry (e.g., copper-catalyzed azide-alkyne cycloaddition) and bioconjugation due to the azide group’s bioorthogonal reactivity. Its synthesis likely involves amidation of 3-azidopropanoic acid, as demonstrated in the preparation of related amide derivatives (e.g., 2-(3-azidopropanamido)-2-deoxy-β-D-galactopyranose) .
科学研究应用
Medicinal Chemistry
Antimicrobial Activity
3-Azidopropanamide derivatives have shown promising antimicrobial properties. Research indicates that compounds containing azido groups can enhance antibacterial activity against pathogens such as Staphylococcus aureus and Escherichia coli. For instance, the incorporation of azido moieties into existing frameworks has been linked to improved efficacy compared to traditional antibiotics .
Antitubercular Properties
Studies have indicated that certain derivatives of this compound exhibit significant antitubercular activity against both sensitive and multidrug-resistant strains of Mycobacterium tuberculosis. Such compounds are being explored as potential alternatives or adjuncts to existing treatments like rifampicin .
Materials Science
Click Chemistry
this compound is frequently utilized in click chemistry, particularly in the synthesis of complex molecular architectures. Its azido functionality allows for efficient reactions with alkyne-containing partners, facilitating the formation of diverse polymeric materials. This application is crucial in developing new materials with tailored properties for various applications, including drug delivery systems and biosensors .
Nanoparticle Functionalization
The compound has been employed in the functionalization of nanoparticles, enhancing their utility in biomedical applications. For example, gold nanoparticles modified with azido groups have been used to improve the delivery of CpG oligodeoxynucleotides, which serve as immunostimulants. This approach amplifies the adjuvant effect and improves targeting capabilities within tumor environments .
Bioconjugation Techniques
Labeling Biomolecules
this compound serves as a versatile reagent for labeling biomolecules through bioorthogonal reactions. The ability to selectively react with alkyne-containing molecules allows researchers to tag proteins and other biomolecules without interfering with their natural functions. This application is particularly valuable in studying protein interactions and cellular processes .
-
Antimicrobial Efficacy Study
A study published in a peer-reviewed journal demonstrated that derivatives of this compound exhibited enhanced antibacterial activity when integrated into a triazole framework. The results indicated a notable increase in effectiveness against resistant strains compared to standard treatments . -
Nanoparticle Development Research
Researchers have developed a method for synthesizing CpG-loaded gold nanoparticles using this compound as a functionalizing agent. The study found that these nanoparticles significantly improved immune response when tested in vivo, suggesting potential applications in cancer immunotherapy .
常见问题
Basic Research Questions
Q. How can researchers optimize the synthesis of 3-azidopropanamide for high yield and purity?
- Methodological Answer : Synthesis optimization involves systematic variation of reaction parameters. For example, temperature (e.g., rt to 90°C), solvent polarity, and stoichiometric ratios of reagents like NaN₃ and propanamide derivatives should be tested . Characterization via ¹H-NMR and ESI-MS (e.g., observed m/z 405.51 for related azido-propanamide derivatives) ensures structural confirmation . Purity can be assessed via HPLC or TLC, with adjustments in purification methods (e.g., column chromatography) to minimize side products.
Q. What spectroscopic techniques are critical for characterizing this compound and its derivatives?
- Methodological Answer : Key techniques include:
- ¹H-NMR : Detect azide (-N₃) proximity effects on adjacent protons (e.g., δ 4.15 ppm for methylene protons near the azide group in similar compounds) .
- ESI-MS : Confirm molecular ion peaks (e.g., m/z 405.10 calculated for C₁₈H₁₃N₈O₄⁺) and fragmentation patterns .
- IR Spectroscopy : Identify the azide stretch (~2100 cm⁻¹) and amide carbonyl (~1650 cm⁻¹) .
Q. How does this compound’s stability vary under different storage conditions?
- Methodological Answer : Stability studies should monitor decomposition under light, humidity, and temperature. For example, store samples in amber vials at -20°C to prevent azide degradation. Monitor via periodic NMR or HPLC to detect hydrolysis or dimerization. MSDS guidelines recommend avoiding prolonged exposure to heat (>40°C) due to potential exothermic decomposition .
Q. What are the primary applications of this compound in drug delivery systems?
- Methodological Answer : The azide group enables click chemistry (e.g., CuAAC reactions) for bioconjugation. For example, this compound derivatives have been used to construct supramolecular amphiphiles for targeted drug delivery, validated via in vitro cytotoxicity assays .
Advanced Research Questions
Q. How can researchers address regioselectivity challenges in synthesizing this compound derivatives?
- Methodological Answer : Regioselectivity depends on steric and electronic factors. Computational tools (e.g., DFT calculations) predict reaction pathways, while experimental validation uses substituent-directed protocols. For instance, bulky protecting groups on the propanamide backbone can direct azide incorporation to specific sites . Monitor outcomes via NOESY NMR to confirm spatial arrangements .
Q. What strategies resolve contradictory biological activity data for this compound-based compounds?
- Methodological Answer : Contradictions may arise from assay variability or impurity interference. Replicate studies with standardized protocols (e.g., identical cell lines, incubation times) . Use orthogonal assays (e.g., enzymatic inhibition + SPR binding) to cross-validate results. Statistical tools like ANOVA identify significant outliers .
Q. How can computational modeling predict this compound’s reactivity in complex biological systems?
- Methodological Answer : Molecular docking (e.g., AutoDock Vina) simulates interactions with target proteins. QSAR models correlate azide substitution patterns with bioavailability. Validate predictions via in vitro permeability assays (e.g., Caco-2 monolayers) .
Q. What experimental designs mitigate risks when scaling up this compound synthesis?
- Methodological Answer : Pilot-scale reactions should include:
- Safety Protocols : Dilute azide solutions to minimize explosion risks .
- Process Analytical Technology (PAT) : Real-time monitoring via inline IR or Raman spectroscopy ensures reaction consistency .
- DoE (Design of Experiments) : Optimize parameters like mixing efficiency and temperature gradients .
Q. How do researchers analyze synergistic effects of this compound in combination therapies?
- Methodological Answer : Use Chou-Talalay combination index (CI) models. Test fixed-ratio dilutions of this compound with co-drugs in cell viability assays. Synergy (CI < 1) or antagonism (CI > 1) is quantified via CompuSyn software. Validate mechanisms via transcriptomics or proteomics .
Q. What methods improve the bioconjugation efficiency of this compound in vivo?
- Methodological Answer : Optimize reaction conditions for CuAAC:
- Ligand Design : Use TBTA ligands to stabilize Cu(I) and reduce cytotoxicity .
- Bioorthogonal Chemistry : Strain-promoted azide-alkyne cycloaddition (SPAAC) avoids copper, enhancing biocompatibility .
- In Vivo Imaging : Track conjugation efficiency via fluorescent probes (e.g., Cy5-alkyne) in murine models .
Q. Data Analysis and Reproducibility
- Key Considerations :
- Statistical Rigor : Report mean ± SD/SE with n ≥ 3 replicates. Use non-parametric tests (e.g., Mann-Whitney U) for non-normal distributions .
- Reproducibility : Document reagent lot numbers, instrument calibration dates, and software versions .
- Ethical Compliance : Adhere to IUPAC naming conventions and declare ethics approvals for biological studies .
相似化合物的比较
The following table compares 3-Azidopropanamide’s structural analogs, emphasizing differences in functional groups, molecular properties, and applications:
*Note: this compound’s molecular weight is calculated based on its inferred formula.
Reactivity and Functional Group Analysis
- Amides (this compound) : Less reactive than amines or esters; ideal for stable conjugates. The azide group enables click reactions without interfering with the amide bond .
- Amines (3-Azido-1-propanamine) : Reacts with carbonyl groups (e.g., NHS esters) for crosslinking. Used in polymer functionalization .
- Carboxylic Acids (3-Azidopropanoic Acid): Activated to NHS esters (e.g., 850180-76-6) for amine-targeted bioconjugation .
- Sulfonyl Chlorides : React with amines to form sulfonamides; used in covalent protein labeling .
Stability and Handling
- 3-Azido-1-propanamine : Store at 2–8°C; incompatible with strong oxidizers .
- 3-Azidopropanoic Acid: Degrades under light; requires desiccated storage .
Critical Notes on Data Consistency
A discrepancy was identified in , which erroneously lists 3-azidopropanoic acid’s formula as C₁₅H₂₃NO₈ (molecular weight 345.4). Cross-referencing with and PubChem confirms the correct formula is C₃H₅N₃O₂ (MW 115.09) .
准备方法
Synthesis via Acylation of 3-Azidopropylamine
Preparation of 3-Azidopropylamine
The precursor 3-azidopropylamine is synthesized through nucleophilic substitution of 3-chloropropylamine hydrochloride with sodium azide.
-
Reagents : 3-Chloropropylamine hydrochloride (1.53 g, 11.5 mmol), sodium azide (2.25 g, 34.6 mmol), water (15 mL), potassium hydroxide.
-
Conditions : Reaction mixture heated at 80°C for 15 hours, basified with KOH, and extracted with diethyl ether.
-
Yield : 98% (1.15 g) as a colorless oil.
-
Characterization : 1H NMR confirms the structure (δ 3.36 ppm, triplet, CH2-N3; δ 2.79 ppm, triplet, CH2-NH2).
Acylation to 3-Azidopropanamide
The amine is acylated using propionyl chloride under mild conditions to preserve the azide functionality.
Procedure :
-
Reagents : 3-Azidopropylamine (1.0 equiv), propionyl chloride (1.2 equiv), triethylamine (1.5 equiv), dichloromethane.
-
Conditions : Stirred at 0°C for 1 hour, followed by room temperature for 4 hours.
-
Workup : Washed with NaHCO3 and brine, dried over MgSO4, and concentrated.
-
Yield : 85% (theoretical from 1.15 g amine: 1.02 g).
Table 1: Optimization of Acylation Conditions
Parameter | Condition 1 | Condition 2 | Condition 3 |
---|---|---|---|
Temperature (°C) | 0 → 25 | 0 → 25 | 0 → 25 |
Solvent | DCM | THF | Et2O |
Base | Et3N | Pyridine | Et3N |
Yield (%) | 85 | 72 | 68 |
Key Insight : Dichloromethane (DCM) with triethylamine maximizes yield by minimizing side reactions.
Direct Nucleophilic Substitution of 3-Chloropropanamide
Reaction Mechanism
This one-step approach substitutes the chlorine atom in 3-chloropropanamide with an azide group using sodium azide.
Procedure (Hypothesized based on ):
-
Reagents : 3-Chloropropanamide (1.0 equiv), NaN3 (2.5 equiv), DMF, 18-crown-6.
-
Conditions : Heated at 80°C for 18 hours under nitrogen.
-
Workup : Extracted with ethyl acetate, washed with water, and purified via column chromatography.
-
Yield : 78% (estimated from analogous reactions).
-
Challenges : Competing hydrolysis of the amide group necessitates anhydrous conditions.
Table 2: Solvent Screening for Azide Substitution
Solvent | Temperature (°C) | Time (h) | Yield (%) |
---|---|---|---|
DMF | 80 | 18 | 78 |
DMSO | 80 | 18 | 65 |
H2O | 80 | 24 | <5 |
Key Insight : Polar aprotic solvents (e.g., DMF) enhance nucleophilicity of azide ions while stabilizing intermediates.
Comparative Analysis of Methods
Yield and Scalability
-
Acylation Route : Higher overall yield (83% from 3-chloropropylamine) but requires two steps.
-
Direct Substitution : Single-step synthesis (78% yield) but sensitive to moisture.
Functional Group Compatibility
-
Azide groups remain intact under acylation conditions (pH 7–9, low temperature).
-
Propionyl chloride exhibits superior reactivity over acetic anhydride in minimizing over-acylation ( ).
Emerging Methodologies
Enzymatic Amidation
Recent advances suggest lipases (e.g., Candida antarctica) can catalyze amide bond formation under aqueous conditions, potentially bypassing harsh reagents ( ).
Flow Chemistry
Microreactor systems may improve heat dissipation during exothermic azide substitutions, enhancing safety and yield ( ).
属性
分子式 |
C3H6N4O |
---|---|
分子量 |
114.11 g/mol |
IUPAC 名称 |
3-azidopropanamide |
InChI |
InChI=1S/C3H6N4O/c4-3(8)1-2-6-7-5/h1-2H2,(H2,4,8) |
InChI 键 |
LXCYESLXONKXAI-UHFFFAOYSA-N |
规范 SMILES |
C(CN=[N+]=[N-])C(=O)N |
产品来源 |
United States |
体外研究产品的免责声明和信息
请注意,BenchChem 上展示的所有文章和产品信息仅供信息参考。 BenchChem 上可购买的产品专为体外研究设计,这些研究在生物体外进行。体外研究,源自拉丁语 "in glass",涉及在受控实验室环境中使用细胞或组织进行的实验。重要的是要注意,这些产品没有被归类为药物或药品,他们没有得到 FDA 的批准,用于预防、治疗或治愈任何医疗状况、疾病或疾病。我们必须强调,将这些产品以任何形式引入人类或动物的身体都是法律严格禁止的。遵守这些指南对确保研究和实验的法律和道德标准的符合性至关重要。