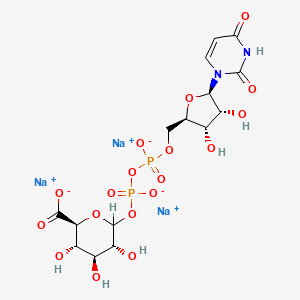
Udpga 3Na
描述
准备方法
Synthetic Routes and Reaction Conditions
The synthesis of uridine 5’-diphosphoglucuronic acid trisodium salt can be achieved through a cascade synthesis route. This involves coupling multiple whole cells expressing hyperthermophilic enzymes to produce uridine 5’-diphosphoglucuronic acid from starch. The process includes a coenzyme regeneration system with an appropriate expression level of uridine diphosphate-glucose 6-dehydrogenase in a single strain, meeting the nicotinamide adenine dinucleotide requirements .
Industrial Production Methods
For industrial production, the cascade synthesis route is scaled up to produce uridine 5’-diphosphoglucuronic acid in large quantities. This method is cost-effective and efficient, yielding high purity uridine 5’-diphosphoglucuronic acid suitable for various applications .
化学反应分析
Types of Reactions
Uridine 5’-diphosphoglucuronic acid trisodium salt undergoes glucuronidation reactions, which are catalyzed by uridine diphosphate glucuronosyltransferases. These reactions involve the covalent linkage of glucuronic acid to a nucleophilic functional group on a typically lipophilic substrate .
Common Reagents and Conditions
The glucuronidation reactions require the presence of uridine 5’-diphosphoglucuronic acid as a cofactor and a nucleophilic substrate. The reactions typically proceed under physiological conditions, with the enzyme uridine diphosphate glucuronosyltransferase facilitating the transfer of glucuronic acid .
Major Products
The major products of these reactions are glucuronides, which are more water-soluble and can be easily excreted from the body. This process is essential for the metabolism and clearance of many lipophilic chemicals, including drugs and endogenous compounds .
科学研究应用
Uridine 5’-diphosphoglucuronic acid trisodium salt has numerous applications in scientific research:
作用机制
Uridine 5’-diphosphoglucuronic acid trisodium salt exerts its effects through glucuronidation, a process catalyzed by uridine diphosphate glucuronosyltransferases. The enzyme facilitates the transfer of glucuronic acid to a nucleophilic functional group on a substrate, forming a glucuronide. This reaction increases the water solubility of the substrate, allowing for its excretion from the body .
相似化合物的比较
Uridine 5’-diphosphoglucuronic acid trisodium salt is unique in its role as a glycosyl donor for glucuronidation reactions. Similar compounds include:
Uridine diphosphate glucose: A precursor in the biosynthesis of uridine 5’-diphosphoglucuronic acid.
Uridine diphosphate galactose: Involved in the biosynthesis of glycoproteins and glycolipids.
Uridine diphosphate N-acetylglucosamine: Participates in the biosynthesis of glycosaminoglycans and glycoproteins.
Uridine 5’-diphosphoglucuronic acid trisodium salt stands out due to its specific role in glucuronidation, a critical pathway for the detoxification and excretion of various compounds .
属性
IUPAC Name |
trisodium;(2S,3S,4S,5R)-6-[[[(2R,3S,4R,5R)-5-(2,4-dioxopyrimidin-1-yl)-3,4-dihydroxyoxolan-2-yl]methoxy-oxidophosphoryl]oxy-oxidophosphoryl]oxy-3,4,5-trihydroxyoxane-2-carboxylate | |
---|---|---|
Details | Computed by Lexichem TK 2.7.0 (PubChem release 2021.05.07) | |
Source | PubChem | |
URL | https://pubchem.ncbi.nlm.nih.gov | |
Description | Data deposited in or computed by PubChem | |
InChI |
InChI=1S/C15H22N2O18P2.3Na/c18-5-1-2-17(15(26)16-5)12-9(22)6(19)4(32-12)3-31-36(27,28)35-37(29,30)34-14-10(23)7(20)8(21)11(33-14)13(24)25;;;/h1-2,4,6-12,14,19-23H,3H2,(H,24,25)(H,27,28)(H,29,30)(H,16,18,26);;;/q;3*+1/p-3/t4-,6-,7+,8+,9-,10-,11+,12-,14?;;;/m1.../s1 | |
Details | Computed by InChI 1.0.6 (PubChem release 2021.05.07) | |
Source | PubChem | |
URL | https://pubchem.ncbi.nlm.nih.gov | |
Description | Data deposited in or computed by PubChem | |
InChI Key |
XXXUNWUNTOMVIG-FBPUXPPNSA-K | |
Details | Computed by InChI 1.0.6 (PubChem release 2021.05.07) | |
Source | PubChem | |
URL | https://pubchem.ncbi.nlm.nih.gov | |
Description | Data deposited in or computed by PubChem | |
Canonical SMILES |
C1=CN(C(=O)NC1=O)C2C(C(C(O2)COP(=O)([O-])OP(=O)([O-])OC3C(C(C(C(O3)C(=O)[O-])O)O)O)O)O.[Na+].[Na+].[Na+] | |
Details | Computed by OEChem 2.3.0 (PubChem release 2021.05.07) | |
Source | PubChem | |
URL | https://pubchem.ncbi.nlm.nih.gov | |
Description | Data deposited in or computed by PubChem | |
Isomeric SMILES |
C1=CN(C(=O)NC1=O)[C@H]2[C@@H]([C@@H]([C@H](O2)COP(=O)([O-])OP(=O)([O-])OC3[C@@H]([C@H]([C@@H]([C@H](O3)C(=O)[O-])O)O)O)O)O.[Na+].[Na+].[Na+] | |
Details | Computed by OEChem 2.3.0 (PubChem release 2021.05.07) | |
Source | PubChem | |
URL | https://pubchem.ncbi.nlm.nih.gov | |
Description | Data deposited in or computed by PubChem | |
Molecular Formula |
C15H19N2Na3O18P2 | |
Details | Computed by PubChem 2.1 (PubChem release 2021.05.07) | |
Source | PubChem | |
URL | https://pubchem.ncbi.nlm.nih.gov | |
Description | Data deposited in or computed by PubChem | |
Molecular Weight |
646.23 g/mol | |
Details | Computed by PubChem 2.1 (PubChem release 2021.05.07) | |
Source | PubChem | |
URL | https://pubchem.ncbi.nlm.nih.gov | |
Description | Data deposited in or computed by PubChem | |
Retrosynthesis Analysis
AI-Powered Synthesis Planning: Our tool employs the Template_relevance Pistachio, Template_relevance Bkms_metabolic, Template_relevance Pistachio_ringbreaker, Template_relevance Reaxys, Template_relevance Reaxys_biocatalysis model, leveraging a vast database of chemical reactions to predict feasible synthetic routes.
One-Step Synthesis Focus: Specifically designed for one-step synthesis, it provides concise and direct routes for your target compounds, streamlining the synthesis process.
Accurate Predictions: Utilizing the extensive PISTACHIO, BKMS_METABOLIC, PISTACHIO_RINGBREAKER, REAXYS, REAXYS_BIOCATALYSIS database, our tool offers high-accuracy predictions, reflecting the latest in chemical research and data.
Strategy Settings
Precursor scoring | Relevance Heuristic |
---|---|
Min. plausibility | 0.01 |
Model | Template_relevance |
Template Set | Pistachio/Bkms_metabolic/Pistachio_ringbreaker/Reaxys/Reaxys_biocatalysis |
Top-N result to add to graph | 6 |
Feasible Synthetic Routes
体外研究产品的免责声明和信息
请注意,BenchChem 上展示的所有文章和产品信息仅供信息参考。 BenchChem 上可购买的产品专为体外研究设计,这些研究在生物体外进行。体外研究,源自拉丁语 "in glass",涉及在受控实验室环境中使用细胞或组织进行的实验。重要的是要注意,这些产品没有被归类为药物或药品,他们没有得到 FDA 的批准,用于预防、治疗或治愈任何医疗状况、疾病或疾病。我们必须强调,将这些产品以任何形式引入人类或动物的身体都是法律严格禁止的。遵守这些指南对确保研究和实验的法律和道德标准的符合性至关重要。