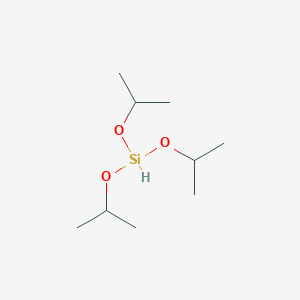
Tris(propan-2-yloxy)silane
描述
C9H22O3Si . This compound is characterized by its three isopropoxy groups attached to a central silicon atom. It is a colorless liquid with a density of approximately 0.8565 g/cm³ and a boiling point of 35°C at 5 mmHg .
准备方法
Synthetic Routes and Reaction Conditions: Tris(propan-2-yloxy)silane is typically synthesized by reacting trichloromethylsilane with isopropanol (2-propanol). The reaction is carried out under an inert atmosphere, such as nitrogen or argon, to prevent moisture from interfering with the reaction. The trichloromethylsilane is slowly added dropwise to isopropanol, and the reaction mixture is maintained at a temperature between room temperature and 60°C. After the reaction is complete, the product is purified through distillation, reduced pressure, or extraction methods .
Industrial Production Methods: The industrial production of this compound follows a similar synthetic route but on a larger scale. The reaction conditions are optimized to ensure high yield and purity of the product. The use of advanced distillation techniques and inert gas environments are crucial to maintaining the quality of the compound .
化学反应分析
Types of Reactions: Tris(propan-2-yloxy)silane undergoes various chemical reactions, including:
Hydrolysis: Reacts slowly with moisture or water to form silanols and isopropanol.
Oxidation: Can be oxidized under specific conditions to form siloxanes.
Substitution: Can participate in substitution reactions where the isopropoxy groups are replaced by other functional groups.
Common Reagents and Conditions:
Hydrolysis: Typically occurs in the presence of water or moisture.
Oxidation: Requires oxidizing agents such as hydrogen peroxide or ozone.
Substitution: Involves reagents like halogens or other nucleophiles.
Major Products:
Hydrolysis: Produces silanols and isopropanol.
Oxidation: Forms siloxanes.
Substitution: Results in various substituted silanes depending on the reagents used.
科学研究应用
Tris(propan-2-yloxy)silane has a wide range of applications in scientific research, including:
Chemistry: Used as a reagent in organic synthesis, particularly in the formation of silicon-containing compounds.
Biology: Employed in the modification of biomolecules and surfaces to enhance their properties.
Medicine: Investigated for its potential use in drug delivery systems and as a component in medical coatings.
Industry: Utilized as a raw material in the production of surfactants and lubricants.
作用机制
The mechanism of action of tris(propan-2-yloxy)silane involves its ability to form strong bonds with various substrates through its silicon atom. The isopropoxy groups can be hydrolyzed to form silanols, which can further condense to form siloxane bonds. This property makes it useful in surface modification and the formation of stable silicon-containing compounds .
相似化合物的比较
Triisopropylsilane: Similar in structure but with different functional groups.
Triethoxysilane: Contains ethoxy groups instead of isopropoxy groups.
Trimethoxysilane: Contains methoxy groups instead of isopropoxy groups.
Uniqueness: Tris(propan-2-yloxy)silane is unique due to its specific isopropoxy groups, which provide distinct reactivity and properties compared to other similar silanes. Its ability to form stable siloxane bonds and its use in various applications make it a valuable compound in both research and industry .
生物活性
Tris(propan-2-yloxy)silane, a silane compound, has garnered attention in various fields of research due to its unique chemical properties and potential biological applications. This article delves into its biological activity, mechanisms of action, and relevant case studies, supported by data tables and research findings.
Chemical Structure:
this compound consists of a silicon atom bonded to three isopropoxy groups. Its ability to form stable siloxane bonds through hydrolysis makes it a valuable reagent in organic synthesis and surface modification.
Mechanism of Action:
The biological activity of this compound is primarily attributed to its capacity to modify biomolecules and surfaces. Upon hydrolysis, it generates silanols that can condense to form siloxanes, enhancing the stability and functionality of modified surfaces or compounds in biological contexts.
Biological Applications
-
Surface Modification:
- This compound is utilized for modifying surfaces to improve biocompatibility and adhesion properties in biomedical devices. This modification enhances the interaction between the device and biological tissues.
-
Drug Delivery Systems:
- Research indicates potential applications in drug delivery, where the compound can be used as a carrier for therapeutic agents, enhancing their stability and bioavailability.
-
Biological Coatings:
- The compound is investigated for use in medical coatings that require enhanced resistance to biological degradation and improved interaction with biological systems.
Case Studies
Case Study 1: Surface Modification for Biomedical Applications
A study demonstrated the effectiveness of this compound in modifying titanium surfaces used in dental implants. The treated surfaces exhibited improved cell adhesion and proliferation when compared to untreated controls, indicating enhanced biocompatibility .
Case Study 2: Drug Delivery Systems
In another investigation, this compound was incorporated into polymeric matrices for controlled drug release. The results showed a sustained release profile for the drug over an extended period, suggesting its potential as a drug delivery vehicle .
Table 1: Summary of Biological Activities
Application | Description | Findings |
---|---|---|
Surface Modification | Enhances biocompatibility of implants | Improved cell adhesion on treated surfaces |
Drug Delivery | Used as a carrier for therapeutic agents | Sustained release profile observed |
Medical Coatings | Provides resistance against biological degradation | Enhanced interaction with biological tissues |
属性
IUPAC Name |
tri(propan-2-yloxy)silane | |
---|---|---|
Details | Computed by LexiChem 2.6.6 (PubChem release 2019.06.18) | |
Source | PubChem | |
URL | https://pubchem.ncbi.nlm.nih.gov | |
Description | Data deposited in or computed by PubChem | |
InChI |
InChI=1S/C9H22O3Si/c1-7(2)10-13(11-8(3)4)12-9(5)6/h7-9,13H,1-6H3 | |
Details | Computed by InChI 1.0.5 (PubChem release 2019.06.18) | |
Source | PubChem | |
URL | https://pubchem.ncbi.nlm.nih.gov | |
Description | Data deposited in or computed by PubChem | |
InChI Key |
JGABXROLARSPEN-UHFFFAOYSA-N | |
Details | Computed by InChI 1.0.5 (PubChem release 2019.06.18) | |
Source | PubChem | |
URL | https://pubchem.ncbi.nlm.nih.gov | |
Description | Data deposited in or computed by PubChem | |
Canonical SMILES |
CC(C)O[SiH](OC(C)C)OC(C)C | |
Details | Computed by OEChem 2.1.5 (PubChem release 2019.06.18) | |
Source | PubChem | |
URL | https://pubchem.ncbi.nlm.nih.gov | |
Description | Data deposited in or computed by PubChem | |
Molecular Formula |
C9H22O3Si | |
Details | Computed by PubChem 2.1 (PubChem release 2019.06.18) | |
Source | PubChem | |
URL | https://pubchem.ncbi.nlm.nih.gov | |
Description | Data deposited in or computed by PubChem | |
Molecular Weight |
206.35 g/mol | |
Details | Computed by PubChem 2.1 (PubChem release 2021.05.07) | |
Source | PubChem | |
URL | https://pubchem.ncbi.nlm.nih.gov | |
Description | Data deposited in or computed by PubChem | |
Retrosynthesis Analysis
AI-Powered Synthesis Planning: Our tool employs the Template_relevance Pistachio, Template_relevance Bkms_metabolic, Template_relevance Pistachio_ringbreaker, Template_relevance Reaxys, Template_relevance Reaxys_biocatalysis model, leveraging a vast database of chemical reactions to predict feasible synthetic routes.
One-Step Synthesis Focus: Specifically designed for one-step synthesis, it provides concise and direct routes for your target compounds, streamlining the synthesis process.
Accurate Predictions: Utilizing the extensive PISTACHIO, BKMS_METABOLIC, PISTACHIO_RINGBREAKER, REAXYS, REAXYS_BIOCATALYSIS database, our tool offers high-accuracy predictions, reflecting the latest in chemical research and data.
Strategy Settings
Precursor scoring | Relevance Heuristic |
---|---|
Min. plausibility | 0.01 |
Model | Template_relevance |
Template Set | Pistachio/Bkms_metabolic/Pistachio_ringbreaker/Reaxys/Reaxys_biocatalysis |
Top-N result to add to graph | 6 |
Feasible Synthetic Routes
体外研究产品的免责声明和信息
请注意,BenchChem 上展示的所有文章和产品信息仅供信息参考。 BenchChem 上可购买的产品专为体外研究设计,这些研究在生物体外进行。体外研究,源自拉丁语 "in glass",涉及在受控实验室环境中使用细胞或组织进行的实验。重要的是要注意,这些产品没有被归类为药物或药品,他们没有得到 FDA 的批准,用于预防、治疗或治愈任何医疗状况、疾病或疾病。我们必须强调,将这些产品以任何形式引入人类或动物的身体都是法律严格禁止的。遵守这些指南对确保研究和实验的法律和道德标准的符合性至关重要。