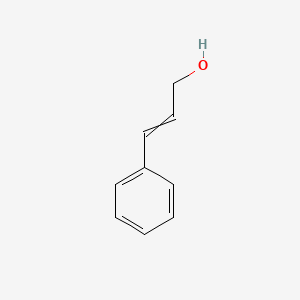
苯乙烯甲醇
概述
描述
Cinnamyl alcohol, also known as cinnamic alcohol or styryl carbinol, is an organic compound with the chemical formula C9H10O. It is a white crystalline solid when pure and a yellow oil when impure. This compound is found in esterified form in storax, Balsam of Peru, and cinnamon leaves . Cinnamyl alcohol is known for its distinctive odor, described as sweet, balsamic, hyacinth, spicy, green, powdery, and cinnamic .
科学研究应用
Cinnamyl alcohol has a wide range of applications in scientific research:
安全和危害
准备方法
Synthetic Routes and Reaction Conditions
Cinnamyl alcohol can be synthesized through several methods. One common method involves the reduction of cinnamaldehyde. This can be achieved using various reducing agents such as sodium borohydride (NaBH4) or catalytic hydrogenation . Another method involves the enzymatic reduction of cinnamaldehyde using carboxylic acid reductase .
Industrial Production Methods
Industrial production of cinnamyl alcohol often involves the chemical synthesis starting from cinnamaldehyde. This process can be optimized using a biphasic system to overcome product inhibition and achieve higher yields . The use of engineered Escherichia coli expressing carboxylic acid reductase has also been explored for the biosynthesis of cinnamyl alcohol .
化学反应分析
Types of Reactions
Cinnamyl alcohol undergoes various chemical reactions, including:
Reduction: It can be reduced to 3-phenyl-1-propanol using hydrogenation catalysts.
Substitution: Cinnamyl alcohol can participate in substitution reactions, such as the Friedel-Crafts alkylation of aromatic compounds.
Common Reagents and Conditions
Oxidation: Potassium permanganate, bimetallic Au–Pd nanoparticles.
Reduction: Sodium borohydride, hydrogenation catalysts.
Substitution: Aluminum-containing mesoporous catalysts.
Major Products
Oxidation: Cinnamaldehyde.
Reduction: 3-phenyl-1-propanol.
Substitution: Alkylated aromatic compounds.
作用机制
The mechanism of action of cinnamyl alcohol involves its interaction with various molecular targets and pathways. It is absorbed through the skin and gut, where it is metabolized to cinnamaldehyde . Cinnamyl alcohol exerts its effects by interacting with cellular membranes, enzymes, and receptors, leading to various biological activities such as antimicrobial and anti-inflammatory effects .
相似化合物的比较
Cinnamyl alcohol is part of the cinnamyl family of compounds, which includes cinnamic aldehyde, cinnamic acid, cinnamic acetate, and 2-methoxy-cinnamaldehyde . Compared to these compounds, cinnamyl alcohol is unique due to its specific odor profile and its use in perfumery and deodorants . Additionally, its role in the biosynthesis of lignin and its potential therapeutic properties make it distinct from other cinnamyl compounds .
Similar Compounds
Cinnamic aldehyde: Known for its sweet and spicy aroma, used in flavoring and fragrances.
Cinnamic acid: Used in the synthesis of
属性
IUPAC Name |
3-phenylprop-2-en-1-ol | |
---|---|---|
Details | Computed by Lexichem TK 2.7.0 (PubChem release 2021.05.07) | |
Source | PubChem | |
URL | https://pubchem.ncbi.nlm.nih.gov | |
Description | Data deposited in or computed by PubChem | |
InChI |
InChI=1S/C9H10O/c10-8-4-7-9-5-2-1-3-6-9/h1-7,10H,8H2 | |
Details | Computed by InChI 1.0.6 (PubChem release 2021.05.07) | |
Source | PubChem | |
URL | https://pubchem.ncbi.nlm.nih.gov | |
Description | Data deposited in or computed by PubChem | |
InChI Key |
OOCCDEMITAIZTP-UHFFFAOYSA-N | |
Details | Computed by InChI 1.0.6 (PubChem release 2021.05.07) | |
Source | PubChem | |
URL | https://pubchem.ncbi.nlm.nih.gov | |
Description | Data deposited in or computed by PubChem | |
Canonical SMILES |
C1=CC=C(C=C1)C=CCO | |
Details | Computed by OEChem 2.3.0 (PubChem release 2021.05.07) | |
Source | PubChem | |
URL | https://pubchem.ncbi.nlm.nih.gov | |
Description | Data deposited in or computed by PubChem | |
Molecular Formula |
C9H10O | |
Details | Computed by PubChem 2.1 (PubChem release 2021.05.07) | |
Source | PubChem | |
URL | https://pubchem.ncbi.nlm.nih.gov | |
Description | Data deposited in or computed by PubChem | |
DSSTOX Substance ID |
DTXSID9041491 | |
Record name | 3-Phenyl-2-propen-1-ol | |
Source | EPA DSSTox | |
URL | https://comptox.epa.gov/dashboard/DTXSID9041491 | |
Description | DSSTox provides a high quality public chemistry resource for supporting improved predictive toxicology. | |
Molecular Weight |
134.17 g/mol | |
Details | Computed by PubChem 2.1 (PubChem release 2021.05.07) | |
Source | PubChem | |
URL | https://pubchem.ncbi.nlm.nih.gov | |
Description | Data deposited in or computed by PubChem | |
Physical Description |
Solid | |
Record name | Cinnamyl alcohol | |
Source | Human Metabolome Database (HMDB) | |
URL | http://www.hmdb.ca/metabolites/HMDB0029697 | |
Description | The Human Metabolome Database (HMDB) is a freely available electronic database containing detailed information about small molecule metabolites found in the human body. | |
Explanation | HMDB is offered to the public as a freely available resource. Use and re-distribution of the data, in whole or in part, for commercial purposes requires explicit permission of the authors and explicit acknowledgment of the source material (HMDB) and the original publication (see the HMDB citing page). We ask that users who download significant portions of the database cite the HMDB paper in any resulting publications. | |
Boiling Point |
250.00 to 258.00 °C. @ 760.00 mm Hg | |
Details | The Good Scents Company Information System | |
Record name | Cinnamyl alcohol | |
Source | Human Metabolome Database (HMDB) | |
URL | http://www.hmdb.ca/metabolites/HMDB0029697 | |
Description | The Human Metabolome Database (HMDB) is a freely available electronic database containing detailed information about small molecule metabolites found in the human body. | |
Explanation | HMDB is offered to the public as a freely available resource. Use and re-distribution of the data, in whole or in part, for commercial purposes requires explicit permission of the authors and explicit acknowledgment of the source material (HMDB) and the original publication (see the HMDB citing page). We ask that users who download significant portions of the database cite the HMDB paper in any resulting publications. | |
CAS No. |
104-54-1 | |
Record name | Cinnamyl alcohol | |
Source | CAS Common Chemistry | |
URL | https://commonchemistry.cas.org/detail?cas_rn=104-54-1 | |
Description | CAS Common Chemistry is an open community resource for accessing chemical information. Nearly 500,000 chemical substances from CAS REGISTRY cover areas of community interest, including common and frequently regulated chemicals, and those relevant to high school and undergraduate chemistry classes. This chemical information, curated by our expert scientists, is provided in alignment with our mission as a division of the American Chemical Society. | |
Explanation | The data from CAS Common Chemistry is provided under a CC-BY-NC 4.0 license, unless otherwise stated. | |
Record name | 3-Phenyl-2-propen-1-ol | |
Source | EPA DSSTox | |
URL | https://comptox.epa.gov/dashboard/DTXSID9041491 | |
Description | DSSTox provides a high quality public chemistry resource for supporting improved predictive toxicology. | |
Record name | Cinnamyl alcohol | |
Source | Human Metabolome Database (HMDB) | |
URL | http://www.hmdb.ca/metabolites/HMDB0029697 | |
Description | The Human Metabolome Database (HMDB) is a freely available electronic database containing detailed information about small molecule metabolites found in the human body. | |
Explanation | HMDB is offered to the public as a freely available resource. Use and re-distribution of the data, in whole or in part, for commercial purposes requires explicit permission of the authors and explicit acknowledgment of the source material (HMDB) and the original publication (see the HMDB citing page). We ask that users who download significant portions of the database cite the HMDB paper in any resulting publications. | |
Melting Point |
33 °C | |
Record name | Cinnamyl alcohol | |
Source | Human Metabolome Database (HMDB) | |
URL | http://www.hmdb.ca/metabolites/HMDB0029697 | |
Description | The Human Metabolome Database (HMDB) is a freely available electronic database containing detailed information about small molecule metabolites found in the human body. | |
Explanation | HMDB is offered to the public as a freely available resource. Use and re-distribution of the data, in whole or in part, for commercial purposes requires explicit permission of the authors and explicit acknowledgment of the source material (HMDB) and the original publication (see the HMDB citing page). We ask that users who download significant portions of the database cite the HMDB paper in any resulting publications. | |
Synthesis routes and methods I
Procedure details
Synthesis routes and methods II
Procedure details
Synthesis routes and methods III
Procedure details
Synthesis routes and methods IV
Procedure details
Retrosynthesis Analysis
AI-Powered Synthesis Planning: Our tool employs the Template_relevance Pistachio, Template_relevance Bkms_metabolic, Template_relevance Pistachio_ringbreaker, Template_relevance Reaxys, Template_relevance Reaxys_biocatalysis model, leveraging a vast database of chemical reactions to predict feasible synthetic routes.
One-Step Synthesis Focus: Specifically designed for one-step synthesis, it provides concise and direct routes for your target compounds, streamlining the synthesis process.
Accurate Predictions: Utilizing the extensive PISTACHIO, BKMS_METABOLIC, PISTACHIO_RINGBREAKER, REAXYS, REAXYS_BIOCATALYSIS database, our tool offers high-accuracy predictions, reflecting the latest in chemical research and data.
Strategy Settings
Precursor scoring | Relevance Heuristic |
---|---|
Min. plausibility | 0.01 |
Model | Template_relevance |
Template Set | Pistachio/Bkms_metabolic/Pistachio_ringbreaker/Reaxys/Reaxys_biocatalysis |
Top-N result to add to graph | 6 |
Feasible Synthetic Routes
体外研究产品的免责声明和信息
请注意,BenchChem 上展示的所有文章和产品信息仅供信息参考。 BenchChem 上可购买的产品专为体外研究设计,这些研究在生物体外进行。体外研究,源自拉丁语 "in glass",涉及在受控实验室环境中使用细胞或组织进行的实验。重要的是要注意,这些产品没有被归类为药物或药品,他们没有得到 FDA 的批准,用于预防、治疗或治愈任何医疗状况、疾病或疾病。我们必须强调,将这些产品以任何形式引入人类或动物的身体都是法律严格禁止的。遵守这些指南对确保研究和实验的法律和道德标准的符合性至关重要。