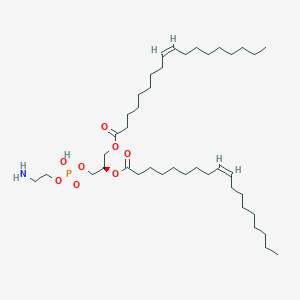
DOPE
概述
描述
DOPE is a neutral phospholipid containing 18:1 fatty acids at the sn-1 and sn-2 positions. This compound is sensitive to pH changes, forming an inverted hexagonal micelle at acidic pH and transforming into a spherical micelle at alkaline pH . It is widely used in various scientific research applications due to its unique properties.
作用机制
Target of Action
DOPE is a metabolite of Escherichia coli with a structure containing 18:1 fatty acids at the sn-1 and sn-2 positions . It primarily targets the cell membrane, where it acts as an emulsifier to facilitate transmembrane translocation of DNA-liposome complexes .
Mode of Action
This compound interacts with its targets by integrating into the cell membrane and altering its properties. This integration facilitates the transmembrane translocation of DNA-liposome complexes, enhancing the efficiency of DNA transfection studies as a method of non-viral gene delivery .
Biochemical Pathways
This compound is involved in the phosphatidylethanolamine biosynthetic pathway. Phosphatidylethanolamine (PE) is formed primarily in the reaction of CDP-ethanolamine and diacylglycerol . In high-temperature water, this compound hydrolyzes to give oleic acid and a number of phosphorus-containing products .
Pharmacokinetics
Its metabolism likely involves hydrolysis, as mentioned above .
Result of Action
The primary result of this compound’s action is the facilitation of transmembrane translocation of DNA-liposome complexes . This can enhance the efficiency of DNA transfection studies, making this compound a valuable tool in non-viral gene delivery . Additionally, a study has shown that a similar compound, 1,2-Dilinoleoyl-sn-glycero-3-phosphoethanolamine (DLPhtEtn), ameliorates age-related spatial memory decline, possibly by protecting hippocampal neuronal death .
Action Environment
This compound is a neutral phospholipid and displays sensitivity towards pH change . At acidic pH, it forms an inverted hexagonal micelle and becomes a spherical micelle at alkaline pH . This suggests that the action, efficacy, and stability of this compound can be influenced by the pH of its environment .
生化分析
Biochemical Properties
1,2-Dioleoyl-sn-glycero-3-phosphoethanolamine plays a crucial role in biochemical reactions. It forms heterogenous liposomes with N-[1-(2,3-dioleoyloxy)propyl]-N,N,N-trimethylammonium methylsulfate (DOTAP), which are used as delivery vehicles for therapeutic agents .
Cellular Effects
1,2-Dioleoyl-sn-glycero-3-phosphoethanolamine has significant effects on various types of cells and cellular processes. When combined with DOTAP, it shows high in vitro transfection efficiency, meaning it effectively transfers genes in lab settings .
Molecular Mechanism
At the molecular level, 1,2-Dioleoyl-sn-glycero-3-phosphoethanolamine exerts its effects through binding interactions with biomolecules and changes in gene expression. Each molecule of DOPE consists of a glycerol backbone to which two oleic acid chains are esterified at the sn-1 and sn-2 positions . These oleic acid chains are unsaturated, containing a cis-double bond, which imparts fluidity to lipid bilayers formed with this compound .
Temporal Effects in Laboratory Settings
Over time in laboratory settings, 1,2-Dioleoyl-sn-glycero-3-phosphoethanolamine has been found to decrease fluorescence lifetime and increase acyl-chain order . It is an essential component of cationic liposomes designed to deliver DNA into gliosarcoma and kidney cell lines .
Metabolic Pathways
1,2-Dioleoyl-sn-glycero-3-phosphoethanolamine is involved in the metabolic pathways of phosphatidylethanolamine . Specific enzymes or cofactors that it interacts with, or its effects on metabolic flux or metabolite levels, are not mentioned in the available literature.
准备方法
Synthetic Routes and Reaction Conditions
DOPE is primarily synthesized through the reaction of CDP-ethanolamine with diacylglycerol . The reaction conditions typically involve the use of chloroform, methanol, and water in specific ratios to achieve the desired product.
Industrial Production Methods
In industrial settings, the production of this compound involves large-scale synthesis using similar reaction conditions as in laboratory settings. The compound is often produced in powder or chloroform form and is stored at low temperatures to maintain stability .
化学反应分析
Types of Reactions
DOPE undergoes various chemical reactions, including:
Oxidation: The compound can be oxidized under specific conditions, leading to the formation of oxidized phospholipids.
Reduction: Reduction reactions can modify the fatty acid chains, altering the compound’s properties.
Substitution: Substitution reactions can occur at the phosphate group, leading to the formation of different phospholipid derivatives.
Common Reagents and Conditions
Oxidation: Common reagents include hydrogen peroxide and other oxidizing agents.
Reduction: Reducing agents such as sodium borohydride are used.
Substitution: Various nucleophiles can be used to substitute the phosphate group.
Major Products Formed
Oxidation: Oxidized phospholipids.
Reduction: Reduced phospholipids with modified fatty acid chains.
Substitution: Phospholipid derivatives with different functional groups.
科学研究应用
DOPE is extensively used in scientific research, including:
Chemistry: Used in the preparation of liposomes and giant unilamellar vesicles (GUVs) for studying membrane dynamics.
Biology: Employed in gene therapy as a component of cationic liposomes designed to deliver DNA into cells.
Medicine: Utilized in drug delivery systems to enhance the delivery of therapeutic agents.
相似化合物的比较
Similar Compounds
1,2-Dioleoyl-sn-glycero-3-phosphocholine: Similar in structure but contains a choline group instead of ethanolamine.
L-α-Phosphatidylethanolamine, dioleoyl: Another phosphatidylethanolamine derivative with similar fatty acid chains.
Uniqueness
DOPE is unique due to its sensitivity to pH changes, which allows it to form different micellar structures under varying conditions. This property makes it particularly useful in the preparation of liposomes and other vesicular systems for drug delivery and gene therapy applications .
生物活性
Dioleoylphosphatidylethanolamine (DOPE) is a neutral phospholipid widely recognized for its unique biological activities, particularly in the fields of gene delivery and membrane fusion. This article explores the biological activity of this compound, highlighting its applications in drug delivery systems, transfection efficiency, and its role in various experimental models.
This compound is chemically defined as 1,2-dioleoyl-sn-glycero-3-phosphoethanolamine. Its molecular structure allows it to adopt different conformations depending on environmental conditions, such as pH. At acidic pH, this compound tends to form inverted hexagonal micelles, while at neutral to alkaline pH, it transitions to spherical micelles. This property is crucial for its application in liposomal formulations and gene delivery systems .
Transfection Efficiency
This compound is commonly used as a co-lipid in cationic liposome formulations to enhance transfection efficiency. Studies have demonstrated that when combined with various cationic lipids such as DOTAP or DC-cholesterol, this compound significantly improves the uptake of plasmid DNA (pDNA) into cells. For instance, formulations containing this compound have shown superior transfection rates in lung and salivary epithelial cells compared to formulations lacking this compound .
Table 1: Transfection Efficiency of Various Lipoplex Formulations
Lipid Composition | Cell Type | Transfection Efficiency (%) |
---|---|---|
DOTAP:this compound (1:1) | BHK Cells | 75 |
GAP-DMORIE:DPyPE | C2C12 Cells | 85 |
GAP-DLRIE:this compound | A549 Cells | 70 |
Data derived from various studies on lipoplex formulations .
The mechanism by which this compound enhances transfection involves facilitating the release of pDNA from endosomes after cellular uptake. This compound's fusogenic properties allow it to destabilize endosomal membranes, promoting the escape of genetic material into the cytoplasm. This characteristic is vital for effective gene delivery systems .
Antioxidant and Antibacterial Activities
Recent studies have also explored the antioxidant properties of this compound. In a carotene emulsion model system, this compound exhibited significant antioxidant activity, suggesting potential applications in protecting cells from oxidative stress . Additionally, this compound has demonstrated antibacterial activity with minimum inhibitory concentrations (MIC) against common pathogens such as E. coli and Bacillus subtilis, indicating its potential as a therapeutic agent against bacterial infections .
Case Studies and Research Findings
A notable study investigated the use of this compound in targeted gene delivery via mannosylated cationic liposomes. The results showed enhanced accumulation of genetic material in the liver following intravenous administration, highlighting the efficacy of this compound in facilitating targeted delivery systems . Another study focused on the formulation of liposomes using varying ratios of this compound and cationic lipids, revealing that increasing the proportion of this compound improved liposome stability and reduced particle size, which is beneficial for cellular uptake .
属性
IUPAC Name |
[(2R)-3-[2-aminoethoxy(hydroxy)phosphoryl]oxy-2-[(Z)-octadec-9-enoyl]oxypropyl] (Z)-octadec-9-enoate | |
---|---|---|
Details | Computed by LexiChem 2.6.6 (PubChem release 2019.06.18) | |
Source | PubChem | |
URL | https://pubchem.ncbi.nlm.nih.gov | |
Description | Data deposited in or computed by PubChem | |
InChI |
InChI=1S/C41H78NO8P/c1-3-5-7-9-11-13-15-17-19-21-23-25-27-29-31-33-40(43)47-37-39(38-49-51(45,46)48-36-35-42)50-41(44)34-32-30-28-26-24-22-20-18-16-14-12-10-8-6-4-2/h17-20,39H,3-16,21-38,42H2,1-2H3,(H,45,46)/b19-17-,20-18-/t39-/m1/s1 | |
Details | Computed by InChI 1.0.5 (PubChem release 2019.06.18) | |
Source | PubChem | |
URL | https://pubchem.ncbi.nlm.nih.gov | |
Description | Data deposited in or computed by PubChem | |
InChI Key |
MWRBNPKJOOWZPW-NYVOMTAGSA-N | |
Details | Computed by InChI 1.0.5 (PubChem release 2019.06.18) | |
Source | PubChem | |
URL | https://pubchem.ncbi.nlm.nih.gov | |
Description | Data deposited in or computed by PubChem | |
Canonical SMILES |
CCCCCCCCC=CCCCCCCCC(=O)OCC(COP(=O)(O)OCCN)OC(=O)CCCCCCCC=CCCCCCCCC | |
Details | Computed by OEChem 2.1.5 (PubChem release 2019.06.18) | |
Source | PubChem | |
URL | https://pubchem.ncbi.nlm.nih.gov | |
Description | Data deposited in or computed by PubChem | |
Isomeric SMILES |
CCCCCCCC/C=C\CCCCCCCC(=O)OC[C@H](COP(=O)(O)OCCN)OC(=O)CCCCCCC/C=C\CCCCCCCC | |
Details | Computed by OEChem 2.1.5 (PubChem release 2019.06.18) | |
Source | PubChem | |
URL | https://pubchem.ncbi.nlm.nih.gov | |
Description | Data deposited in or computed by PubChem | |
Molecular Formula |
C41H78NO8P | |
Details | Computed by PubChem 2.1 (PubChem release 2019.06.18) | |
Source | PubChem | |
URL | https://pubchem.ncbi.nlm.nih.gov | |
Description | Data deposited in or computed by PubChem | |
DSSTOX Substance ID |
DTXSID601029609 | |
Record name | 1,2-Dioleoyl-sn-glycero-3-phosphoethanolamine | |
Source | EPA DSSTox | |
URL | https://comptox.epa.gov/dashboard/DTXSID601029609 | |
Description | DSSTox provides a high quality public chemistry resource for supporting improved predictive toxicology. | |
Molecular Weight |
744.0 g/mol | |
Details | Computed by PubChem 2.1 (PubChem release 2021.05.07) | |
Source | PubChem | |
URL | https://pubchem.ncbi.nlm.nih.gov | |
Description | Data deposited in or computed by PubChem | |
Physical Description |
Solid | |
Record name | PE(18:1(9Z)/18:1(9Z)) | |
Source | Human Metabolome Database (HMDB) | |
URL | http://www.hmdb.ca/metabolites/HMDB0009059 | |
Description | The Human Metabolome Database (HMDB) is a freely available electronic database containing detailed information about small molecule metabolites found in the human body. | |
Explanation | HMDB is offered to the public as a freely available resource. Use and re-distribution of the data, in whole or in part, for commercial purposes requires explicit permission of the authors and explicit acknowledgment of the source material (HMDB) and the original publication (see the HMDB citing page). We ask that users who download significant portions of the database cite the HMDB paper in any resulting publications. | |
CAS No. |
4004-05-1 | |
Record name | 1,2-Dioleoyl-sn-glycero-3-phosphoethanolamine | |
Source | CAS Common Chemistry | |
URL | https://commonchemistry.cas.org/detail?cas_rn=4004-05-1 | |
Description | CAS Common Chemistry is an open community resource for accessing chemical information. Nearly 500,000 chemical substances from CAS REGISTRY cover areas of community interest, including common and frequently regulated chemicals, and those relevant to high school and undergraduate chemistry classes. This chemical information, curated by our expert scientists, is provided in alignment with our mission as a division of the American Chemical Society. | |
Explanation | The data from CAS Common Chemistry is provided under a CC-BY-NC 4.0 license, unless otherwise stated. | |
Record name | 1,2-Dioleoyl-sn-glycero-3-phosphoethanolamine | |
Source | ChemIDplus | |
URL | https://pubchem.ncbi.nlm.nih.gov/substance/?source=chemidplus&sourceid=0004004051 | |
Description | ChemIDplus is a free, web search system that provides access to the structure and nomenclature authority files used for the identification of chemical substances cited in National Library of Medicine (NLM) databases, including the TOXNET system. | |
Record name | 1,2-Dioleoyl-sn-glycero-3-phosphoethanolamine | |
Source | EPA DSSTox | |
URL | https://comptox.epa.gov/dashboard/DTXSID601029609 | |
Description | DSSTox provides a high quality public chemistry resource for supporting improved predictive toxicology. | |
Record name | 1,2-DIOLEOYL-SN-GLYCERO-3-PHOSPHOETHANOLAMINE | |
Source | FDA Global Substance Registration System (GSRS) | |
URL | https://gsrs.ncats.nih.gov/ginas/app/beta/substances/JNP6V6AI0U | |
Description | The FDA Global Substance Registration System (GSRS) enables the efficient and accurate exchange of information on what substances are in regulated products. Instead of relying on names, which vary across regulatory domains, countries, and regions, the GSRS knowledge base makes it possible for substances to be defined by standardized, scientific descriptions. | |
Explanation | Unless otherwise noted, the contents of the FDA website (www.fda.gov), both text and graphics, are not copyrighted. They are in the public domain and may be republished, reprinted and otherwise used freely by anyone without the need to obtain permission from FDA. Credit to the U.S. Food and Drug Administration as the source is appreciated but not required. | |
Retrosynthesis Analysis
AI-Powered Synthesis Planning: Our tool employs the Template_relevance Pistachio, Template_relevance Bkms_metabolic, Template_relevance Pistachio_ringbreaker, Template_relevance Reaxys, Template_relevance Reaxys_biocatalysis model, leveraging a vast database of chemical reactions to predict feasible synthetic routes.
One-Step Synthesis Focus: Specifically designed for one-step synthesis, it provides concise and direct routes for your target compounds, streamlining the synthesis process.
Accurate Predictions: Utilizing the extensive PISTACHIO, BKMS_METABOLIC, PISTACHIO_RINGBREAKER, REAXYS, REAXYS_BIOCATALYSIS database, our tool offers high-accuracy predictions, reflecting the latest in chemical research and data.
Strategy Settings
Precursor scoring | Relevance Heuristic |
---|---|
Min. plausibility | 0.01 |
Model | Template_relevance |
Template Set | Pistachio/Bkms_metabolic/Pistachio_ringbreaker/Reaxys/Reaxys_biocatalysis |
Top-N result to add to graph | 6 |
Feasible Synthetic Routes
Q1: What is the molecular formula and weight of DOPE?
A1: The molecular formula of this compound is C41H78NO8P, and its molecular weight is 744.03 g/mol.
Q2: What is unique about the structure of this compound compared to other phospholipids?
A2: Unlike phosphatidylcholine lipids, which have a bulky headgroup, this compound possesses a relatively small ethanolamine headgroup. This structural feature contributes to its tendency to form non-bilayer structures, such as the inverted hexagonal (HII) phase, and influences its interactions with other lipids and molecules. []
Q3: How does this compound influence the properties of lipid bilayers?
A3: this compound can significantly impact the properties of lipid bilayers. For instance, it can promote membrane fusion and destabilization, especially in acidic environments, making it valuable for drug and gene delivery applications. [] Its presence can also induce curvature in bilayers, impacting membrane protein function and influencing the formation of different lipid phases. [, ]
Q4: How does the presence of this compound in lipid mixtures affect their phase behavior?
A4: this compound can dramatically alter the phase behavior of lipid mixtures. In mixtures with other lipids like DOPC, the inclusion of this compound can lead to phase separation, creating domains with distinct compositions and properties. This phase separation can influence the interaction of the lipid mixture with other molecules, like proteins and drugs. []
Q5: What techniques are commonly used to study the structural properties and interactions of this compound?
A5: Researchers utilize various techniques to study this compound, including: * Langmuir balance: To analyze the behavior of this compound monolayers at the air-water interface, providing information on molecular packing and interactions. [] * Synchrotron X-ray diffraction: To determine the structural organization and phase behavior of this compound-containing systems, such as complexes with DNA or other lipids. [, ] * Deuterium (2H) NMR spectroscopy: To investigate the molecular order, dynamics, and interactions of this compound within lipid bilayers and non-bilayer phases. [] * Molecular dynamics (MD) simulations: To model the behavior of this compound in lipid bilayers and study its interactions with other molecules at the atomic level. [, ]
Q6: Why is this compound considered a valuable component in lipid-based drug and gene delivery systems?
A6: this compound's fusogenic properties, particularly in acidic environments like endosomes, make it highly valuable for drug and gene delivery. When incorporated into liposomes or other lipid nanoparticles, this compound can promote the fusion of these carriers with cell membranes, facilitating the release of encapsulated therapeutic agents into the cytoplasm. [, , ]
Q7: How does this compound compare to other helper lipids used in liposomal formulations?
A7: While cholesterol is another widely used helper lipid, this compound often demonstrates superior performance in gene delivery applications. Liposomes containing this compound, compared to cholesterol-containing formulations, have shown higher transfection efficiencies. [] This difference is attributed to this compound's fusogenic properties, facilitating the release of genetic material into the cytoplasm.
Q8: Can you provide an example of a successful application of this compound in a drug delivery system?
A8: Researchers have successfully designed an acid-labile cholesterol-vinyl ether-PEG conjugate that, when incorporated with this compound into liposomes, facilitated targeted cytoplasmic delivery of calcein to folate receptor-positive KB cells. The acidic environment of the endosome triggered the cleavage of the conjugate, exposing the fusogenic this compound and promoting content release. []
Q9: How does the ratio of this compound to other lipids in a liposome affect its properties and efficacy?
A9: The this compound to other lipids ratio in a liposome is crucial for its functionality. Higher this compound concentrations can enhance membrane fusion and destabilize the liposome, potentially leading to premature release of encapsulated drugs or increased cytotoxicity. [, ] Therefore, optimizing this ratio is essential for achieving desired drug delivery outcomes.
Q10: How can the surface of this compound-containing liposomes be modified to improve their targeting ability?
A10: To enhance targeting, researchers can functionalize this compound-containing liposomes by conjugating them with targeting ligands, such as antibodies, peptides, or small molecules. For example, conjugating liposomes with a peptide targeting the α5β1 integrin significantly improved gene delivery to DLD-1 colorectal cancer cells. []
Q11: How does this compound interact with DNA in the formation of lipoplexes?
A11: this compound, when present in cationic liposomes, interacts with DNA through a combination of electrostatic and hydrophobic forces to form lipoplexes. The positively charged headgroups of the cationic lipids interact with the negatively charged phosphate backbone of DNA, while this compound helps with the overall complex stability and contributes to membrane fusion during cellular uptake. [, , ]
Q12: How does the structure of the cationic lipid component in a lipoplex influence its interaction with this compound and DNA?
A12: The structure of the cationic lipid, particularly the length and saturation of its hydrophobic tails, significantly influences lipoplex formation and efficacy. For example, cationic lipids with longer tails (C18 or more) often show better gene silencing activity compared to those with shorter tails, likely due to their ability to better accommodate and organize this compound within the lipoplex structure. []
Q13: Are there any studies investigating the interaction of this compound with other molecules besides DNA?
A13: Yes, studies have explored the interaction of this compound with other molecules, including proteins and small molecules. For instance, research has shown that cholesterol interacts strongly with this compound in mixed monolayers, leading to condensation effects and influencing the overall properties of the monolayer. [] Additionally, research has examined the preferential adsorption of L-histidine to liposomes containing this compound and other lipids, demonstrating the influence of lipid composition on small molecule interactions with membranes. []
Q14: What is known about the safety and biocompatibility of this compound for in vivo applications?
A14: this compound is generally considered biocompatible and well-tolerated in preclinical studies. Research on a cationic derivative of polyprenol, when incorporated into liposomes with this compound, did not show significant adverse effects on renal function in rats, suggesting its potential suitability for drug delivery applications. []
Q15: Are there any known concerns or limitations regarding the use of this compound in drug delivery systems?
A15: While this compound is generally safe, optimizing its concentration in formulations is crucial. High this compound concentrations can potentially increase liposome instability and cytotoxicity, potentially causing adverse effects. [, ] Therefore, careful formulation design and thorough toxicity evaluation are essential for ensuring the safety of this compound-containing drug delivery systems.
体外研究产品的免责声明和信息
请注意,BenchChem 上展示的所有文章和产品信息仅供信息参考。 BenchChem 上可购买的产品专为体外研究设计,这些研究在生物体外进行。体外研究,源自拉丁语 "in glass",涉及在受控实验室环境中使用细胞或组织进行的实验。重要的是要注意,这些产品没有被归类为药物或药品,他们没有得到 FDA 的批准,用于预防、治疗或治愈任何医疗状况、疾病或疾病。我们必须强调,将这些产品以任何形式引入人类或动物的身体都是法律严格禁止的。遵守这些指南对确保研究和实验的法律和道德标准的符合性至关重要。