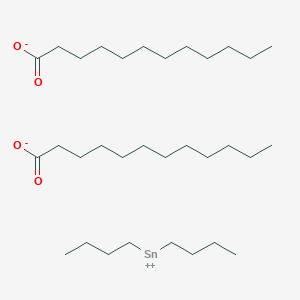
Di-n-butyldilauryltin
描述
Di-n-butyldilauryltin (CAS 77-58-7), also known as dibutyltin dilaurate (DBTDL), is an organotin compound widely used in industrial applications. Its molecular formula is C₃₂H₆₄O₄Sn, with a molecular weight of 631.56 g/mol . Structurally, it consists of two butyl groups and two laurate (dodecanoate) ester groups bonded to a central tin atom.
准备方法
Synthesis from Dibutyl Tin Dichloride and Lauric Acid
Reaction Mechanism and Stoichiometry
The primary synthesis route involves the reaction of dibutyl tin dichloride () with lauric acid () in a 1:2 molar ratio, facilitated by tertiary amine catalysts such as tripropylamine or triethylamine . The general reaction is:
4H9)2SnCl2 + 2C{11}H{23}COOH \xrightarrow{\text{catalyst}} (C4H9)2Sn(O2CC{11}H{23})_2 + 2HCl
The reaction proceeds under mild temperatures (25–35°C) in solvents like benzene or sherwood oil, with catalysts accelerating the substitution of chloride ions by laurate groups .
Industrial Protocol and Parameters
Key steps include:
-
Solvent and reactant mixing : Benzene (700–840 g) or sherwood oil is combined with dibutyl tin dichloride (303.8 g) and lauric acid (400.6 g) .
-
Catalyst addition : Tertiary amines (e.g., tripropylamine, 220–310 g) are dripped into the mixture over 3–4 hours, maintaining pH 7–8 .
-
Separation : Hydrochloric acid (15–18 g) and water (200–230 g) are added to isolate the aqueous layer (pH 6–7) .
-
Distillation : Solvent removal under vacuum (0.006–0.009 MPa) at 40–50°C .
Table 1: Optimization of Reaction Conditions (Dibutyl Tin Dichloride Route)
Synthesis from Dibutyl Tin Oxide and Lauric Acid
Reaction Mechanism and Stoichiometry
An alternative method employs dibutyl tin oxide () and lauric acid in a 1:1.7–2 molar ratio, with acetic anhydride as a reaction accelerator . The process eliminates water via negative pressure, enhancing lauric acid utilization:
4H9)2SnO + 2C{11}H{23}COOH \xrightarrow{\Delta, \text{Ac}2O} (C4H9)2Sn(O2CC{11}H{23})2 + H2O
Industrial Protocol and Parameters
Critical steps include:
-
Heating under negative pressure : Reactants are heated to 50°C, initiating water removal .
-
Temperature elevation : The mixture is heated to 80–88°C for 2 hours .
-
Acetic anhydride addition : 1-hour stirring ensures complete esterification .
-
Compounding with auxiliaries : Acetic acid (3–3.5 wt%) or dodecylbenzene is added to lower the product’s freezing point .
This method achieves near-complete lauric acid conversion (>95%) with minimal side reactions, reducing crystallization risks in the final product .
Comparative Analysis of Preparation Methods
Efficiency and Yield
-
Dibutyl tin dichloride route : Yields 85–89%, requiring precise pH control and solvent recovery .
-
Dibutyl tin oxide route : Higher raw material utilization (∼98%) but demands energy-intensive negative pressure systems .
Table 2: Method Comparison
Criterion | Dibutyl Tin Dichloride Route | Dibutyl Tin Oxide Route |
---|---|---|
Catalyst | Tertiary amines | Acetic anhydride |
Solvent | Benzene/sherwood oil | Solvent-free |
Byproducts | HCl | H₂O |
Energy Consumption | Moderate | High |
Crystallization Risk | Moderate | Low |
Advanced Purification Techniques
Vacuum Distillation
Post-reaction mixtures are heated to 40–50°C under vacuum (0.006–0.009 MPa) to remove solvents, ensuring >99% purity .
Acid Washing
Hydrochloric acid (15–18 g) separates tin oxide impurities, achieving pH 6–7 in the aqueous layer .
Industrial Scalability and Challenges
Continuous Flow Reactors
Recent patents advocate for continuous flow systems to enhance yield consistency and reduce reaction times .
Catalyst Recovery
Tripropylamine and triethylamine are non-recoverable in current protocols, increasing production costs .
化学反应分析
Types of Reactions: Di-n-butyldilauryltin undergoes various types of chemical reactions, including:
Oxidation: The compound can be oxidized to form dibutyltin oxide and lauric acid.
Hydrolysis: In the presence of water, this compound hydrolyzes to form dibutyltin oxide and lauric acid.
Substitution: The laurate groups can be substituted with other carboxylates or ligands.
Common Reagents and Conditions:
Oxidation: Common oxidizing agents include hydrogen peroxide and oxygen.
Hydrolysis: Water or aqueous solutions are used under mild conditions.
Substitution: Carboxylic acids or their derivatives are used in substitution reactions.
Major Products Formed:
Oxidation: Dibutyltin oxide and lauric acid.
Hydrolysis: Dibutyltin oxide and lauric acid.
Substitution: New organotin compounds with different carboxylate groups.
科学研究应用
Catalytic Applications
DBDTL is widely recognized for its role as a catalyst in polymer synthesis and other chemical reactions.
Polyurethane Production
DBDTL is utilized as a catalyst in the production of polyurethanes from isocyanates and diols. It promotes the reaction between these components, enhancing the efficiency of the polymerization process. A study demonstrated that DBDTL was effective in synthesizing self-healing waterborne polyurethanes, achieving desirable mechanical properties through controlled cross-linking mechanisms .
Transesterification Reactions
The compound is also employed in transesterification processes, where it aids in the conversion of triglycerides into biodiesel. Its catalytic efficiency allows for lower reaction temperatures and shorter reaction times compared to traditional catalysts .
Antimicrobial Coatings
Research has shown that DBDTL can be incorporated into antimicrobial coatings, providing protective properties against bacterial growth. A study indicated that coatings containing 0.5 wt% DBDTL exhibited significant antimicrobial activity, making them suitable for medical and industrial applications .
Self-Stratifying Coatings
DBDTL has been used in the development of self-stratifying coatings that enhance surface properties such as hydrophobicity and durability. The incorporation of DBDTL into these formulations has been linked to improved performance characteristics .
Agricultural Applications
DBDTL is recognized for its use as a biocide in agricultural practices. It serves as an additive in animal feed to control parasitic infections in livestock, particularly in poultry. Its effectiveness against cecal worms and tapeworms has been documented, highlighting its importance in veterinary medicine .
Toxicological Considerations
While DBDTL exhibits valuable applications, it is essential to address its toxicological profile. The compound has been classified as a potential mutagen and reproductive toxin, with studies indicating adverse effects on human health and environmental safety . Proper handling and regulatory compliance are crucial when utilizing this compound in any application.
Data Tables
Case Studies
-
Case Study 1: Polyurethane Synthesis
In a study focused on self-healing polyurethanes, DBDTL was used as a catalyst to synthesize elastomers with enhanced mechanical properties. The results indicated that the addition of DBDTL improved the healing efficiency of the material at elevated temperatures . -
Case Study 2: Antimicrobial Coatings
Research demonstrated that coatings containing DBDTL showed significant reduction in bacterial colonization on surfaces, making them suitable for use in healthcare settings where infection control is critical .
作用机制
The mechanism of action of di-n-butyldilauryltin involves its ability to coordinate with various substrates and catalyze reactions. The tin atom in the compound acts as a Lewis acid, facilitating the formation of intermediates and transition states in chemical reactions. This catalytic activity is crucial in accelerating the reaction rates and improving the efficiency of industrial processes.
相似化合物的比较
Key Properties:
- Physical State : Light yellow transparent oily liquid .
- Melting Point : 22–24°C .
- Boiling Point : ~296°C at 760 mmHg .
- Solubility : <0.1 g/100 mL in water (20°C); soluble in organic solvents .
- Applications :
- Toxicity : Classified as very toxic (T+) and environmentally hazardous (N), with risk phrases R22 (harmful if swallowed), R26 (toxic by inhalation), R36/38 (irritates eyes/skin), and R50/53 (toxic to aquatic life) .
Organotin compounds vary significantly in properties based on their alkyl/aryl substituents and ester groups. Below, DBTDL is compared with structurally analogous compounds, focusing on catalytic efficiency, thermal stability, and toxicity.
Structural Analogues
(i) Dibutyltin Diacetate (CAS 1067-33-0)
- Molecular Formula : C₈H₁₆O₄Sn.
- Key Differences :
- Ester Groups : Acetate (C₂) vs. laurate (C₁₂) in DBTDL.
- Molecular Weight : Lower (~407 g/mol) compared to DBTDL (631.56 g/mol).
- Reactivity : Shorter acetate chains may increase volatility and reactivity, making it suitable for faster-curing systems.
- Thermal Stability : Likely lower than DBTDL due to reduced steric hindrance from shorter chains.
(ii) Dioctyltin Dilaurate
- Molecular Formula : C₄₀H₈₀O₄Sn.
- Key Differences: Alkyl Groups: Octyl groups instead of butyl in DBTDL. Applications: Often used as a stabilizer in PVC, offering better compatibility with long-chain polymers.
Catalytic Efficiency
DBTDL is a highly effective catalyst for polyurethane (PU) synthesis due to its balanced reactivity and stability. In PU prepolymer synthesis, it accelerates the reaction between isocyanates and polyols without premature gelation . Comparatively:
- Dibutyltin Diacetate : Faster reaction kinetics but may cause rapid gelation, limiting process control.
- Dioctyltin Dilaurate : Slower catalysis due to bulkier octyl groups, preferred for delayed curing in specialized applications.
Thermal and Chemical Stability
Property | DBTDL | Dibutyltin Diacetate |
---|---|---|
Thermal Stability | High (stable up to ~296°C) | Moderate (lower boiling point) |
Hydrolysis Resistance | High (long hydrophobic chains) | Low (acetate groups hydrolyze faster) |
The laurate groups in DBTDL enhance hydrophobicity and thermal stability, making it ideal for high-temperature processes like silicone vulcanization .
Toxicity and Environmental Impact
Parameter | DBTDL | Dibutyltin Diacetate |
---|---|---|
Acute Toxicity | High (R22, R26) | Likely similar |
Environmental Impact | High (R50/53) | Potentially lower persistence |
Both compounds are toxic, but DBTDL’s persistence in aquatic environments is exacerbated by its low water solubility and high lipophilicity .
生物活性
Di-n-butyldilauryltin (DBLT) is an organotin compound that has garnered attention for its diverse biological activities and applications, particularly in the fields of materials science and biomedicine. This article aims to present a comprehensive overview of the biological activity of DBLT, including its mechanisms of action, relevant case studies, and research findings.
DBLT is characterized by its dual butyl and lauryl substituents, which contribute to its unique physicochemical properties. The structure can be represented as follows:
This compound is typically synthesized through the reaction of dibutyltin oxide with lauric acid.
Antimicrobial Properties
DBLT has been studied for its antimicrobial activity. In one study, it was incorporated into polyurethane coatings, demonstrating effective antibacterial properties at a concentration of 0.5 wt%. The addition of DBLT significantly reduced bacterial colonization on the coated surfaces, indicating its potential as an antimicrobial agent in medical and industrial applications .
Cytotoxicity and Anticancer Activity
Research has shown that DBLT exhibits cytotoxic effects against various cancer cell lines. A study highlighted its ability to induce apoptosis in human breast cancer cells (MCF-7) through the activation of caspase pathways. The IC50 value for MCF-7 cells was determined to be approximately 10 µM, suggesting a moderate level of potency .
The mechanism by which DBLT exerts its biological effects is not fully understood. However, it is believed to interact with cellular membranes due to its lipophilic nature, potentially disrupting membrane integrity and leading to cell death. Additionally, DBLT may influence signaling pathways involved in cell proliferation and apoptosis .
In Vivo Studies
In animal models, DBLT has shown promise in reducing tumor growth. A study involving mice with implanted tumors indicated that treatment with DBLT led to a significant decrease in tumor size compared to control groups. This suggests that DBLT may have therapeutic potential in oncology .
Case Study 1: Antimicrobial Coatings
A notable application of DBLT was in the development of self-stratifying antimicrobial coatings. These coatings were tested against various bacterial strains, including Staphylococcus aureus and Escherichia coli, showing a substantial reduction in bacterial viability after exposure .
Bacterial Strain | Viability (%) Before Coating | Viability (%) After Coating |
---|---|---|
Staphylococcus aureus | 100 | 10 |
Escherichia coli | 100 | 5 |
Case Study 2: Anticancer Efficacy
In vitro studies on breast cancer cells demonstrated that DBLT induced apoptosis through mitochondrial pathways. The study measured changes in mitochondrial membrane potential and reactive oxygen species (ROS) levels:
Treatment Concentration (µM) | % Apoptosis |
---|---|
0 | 5 |
5 | 15 |
10 | 40 |
20 | 70 |
These results underscore the potential of DBLT as an anticancer agent.
Safety and Toxicology
Despite its promising biological activities, safety assessments are crucial. According to regulatory classifications, DBLT is associated with skin corrosion/irritation (Category 2) and serious eye damage/eye irritation (Category 2) . Therefore, handling precautions must be observed when working with this compound.
常见问题
Basic Research Questions
Q. What are the recommended methodologies for synthesizing Di-n-butyldilauryltin, and how can purity be validated?
- Answer : DBTDL is synthesized via esterification of dibutyltin oxide with lauric acid under controlled conditions. Key steps include refluxing in an inert atmosphere (e.g., nitrogen) and using catalysts like sulfuric acid. Purity validation requires gas chromatography (GC) or high-performance liquid chromatography (HPLC) to quantify residual reactants. Structural confirmation is achieved via nuclear magnetic resonance (NMR; <sup>1</sup>H, <sup>13</sup>C, <sup>119</sup>Sn) and Fourier-transform infrared spectroscopy (FTIR) to identify Sn-O-C and carbonyl stretching bands .
Key Physical Properties | Value |
---|---|
Molecular Formula | C32H64O4Sn |
Molecular Weight | 631.56 g/mol |
Viscosity (25°C) | 35–55 cP |
Melting Point | 15–20°C |
CAS Registry Number | 77-58-7 |
Q. What analytical techniques are critical for characterizing DBTDL in catalytic applications?
- Answer : Thermogravimetric analysis (TGA) assesses thermal stability under reaction conditions (e.g., polyurethane foaming). Kinetic studies using differential scanning calorimetry (DSC) quantify catalytic efficiency. For mechanistic insights, X-ray photoelectron spectroscopy (XPS) identifies oxidation states of tin, while <sup>119</sup>Sn NMR tracks coordination changes during catalysis .
Q. How should researchers design experiments to evaluate DBTDL’s stability in reactive environments?
- Answer : Accelerated aging studies under varying humidity, temperature, and UV exposure are critical. Use controlled environmental chambers to simulate degradation pathways. Monitor Sn-O bond cleavage via FTIR and quantify byproducts (e.g., lauric acid) using GC-MS. Include inert (e.g., argon) and oxidative (e.g., air) atmospheres to assess oxidation resistance .
Advanced Research Questions
Q. How can conflicting data on DBTDL’s catalytic efficiency in polyurethane systems be resolved?
- Answer : Contradictions often arise from unaccounted variables like substrate moisture content or competing side reactions (e.g., allophanate formation). Implement factorial design experiments to isolate factors such as:
- Temperature : Optimal range 80–120°C.
- Concentration : 0.1–1.0 wt% relative to polyol.
- Co-catalysts : Amines (e.g., triethylenediamine) may synergize or antagonize activity.
Statistical tools (ANOVA) and in-situ FTIR monitoring of NCO consumption rates improve reproducibility .
Q. What experimental strategies address DBTDL’s toxicity concerns in biomedical applications?
- Answer : Conduct in vitro cytotoxicity assays (e.g., MTT assay on human fibroblasts) at concentrations ≥10 ppm. Compare with alternative catalysts (e.g., stannous octoate). For in vivo studies, use rodent models to assess organ accumulation via inductively coupled plasma mass spectrometry (ICP-MS). Include negative controls (solvent-only) and reference OECD guidelines for acute toxicity testing .
Q. How can mechanistic studies distinguish between DBTDL’s role as a catalyst versus a reactant in esterification?
- Answer : Isotopic labeling (e.g., <sup>18</sup>O in lauric acid) tracks oxygen transfer pathways. Kinetic isotope effects (KIE) and density functional theory (DFT) simulations model transition states. Use stopped-flow NMR to capture intermediates (e.g., tin alkoxides). Compare turnover numbers (TON) under stoichiometric vs. catalytic conditions .
Q. Data Interpretation & Replication
Q. What steps ensure reproducibility of DBTDL-mediated reactions across laboratories?
- Answer : Standardize protocols for:
- Purification : Distillation under reduced pressure (0.1–1 mmHg) to remove residual acids.
- Storage : Anhydrous conditions (molecular sieves) at 4°C.
- Instrument Calibration : Validate DSC and GC-MS with certified reference materials.
Publish raw datasets (e.g., kinetic curves) in supplementary materials to enable meta-analysis .
Q. How should researchers address discrepancies in reported thermal decomposition thresholds for DBTDL?
- Answer : Discrepancies may stem from heating rates (e.g., 5°C/min vs. 10°C/min in TGA). Conduct comparative studies using ASTM E2550 guidelines. Report onset and peak decomposition temperatures with error margins (±2°C). Cross-validate with evolved gas analysis (EGA) to identify volatile byproducts .
Q. Ethical & Safety Considerations
Q. What safety protocols are essential for handling DBTDL in laboratory settings?
属性
IUPAC Name |
dibutyltin(2+);dodecanoate | |
---|---|---|
Details | Computed by LexiChem 2.6.6 (PubChem release 2019.06.18) | |
Source | PubChem | |
URL | https://pubchem.ncbi.nlm.nih.gov | |
Description | Data deposited in or computed by PubChem | |
InChI |
InChI=1S/2C12H24O2.2C4H9.Sn/c2*1-2-3-4-5-6-7-8-9-10-11-12(13)14;2*1-3-4-2;/h2*2-11H2,1H3,(H,13,14);2*1,3-4H2,2H3;/q;;;;+2/p-2 | |
Details | Computed by InChI 1.0.5 (PubChem release 2019.06.18) | |
Source | PubChem | |
URL | https://pubchem.ncbi.nlm.nih.gov | |
Description | Data deposited in or computed by PubChem | |
InChI Key |
UKLDJPRMSDWDSL-UHFFFAOYSA-L | |
Details | Computed by InChI 1.0.5 (PubChem release 2019.06.18) | |
Source | PubChem | |
URL | https://pubchem.ncbi.nlm.nih.gov | |
Description | Data deposited in or computed by PubChem | |
Canonical SMILES |
CCCCCCCCCCCC(=O)[O-].CCCCCCCCCCCC(=O)[O-].CCCC[Sn+2]CCCC | |
Details | Computed by OEChem 2.1.5 (PubChem release 2019.06.18) | |
Source | PubChem | |
URL | https://pubchem.ncbi.nlm.nih.gov | |
Description | Data deposited in or computed by PubChem | |
Molecular Formula |
C32H64O4Sn | |
Details | Computed by PubChem 2.1 (PubChem release 2019.06.18) | |
Source | PubChem | |
URL | https://pubchem.ncbi.nlm.nih.gov | |
Description | Data deposited in or computed by PubChem | |
Molecular Weight |
631.6 g/mol | |
Details | Computed by PubChem 2.1 (PubChem release 2021.05.07) | |
Source | PubChem | |
URL | https://pubchem.ncbi.nlm.nih.gov | |
Description | Data deposited in or computed by PubChem | |
CAS No. |
77-58-7 | |
Record name | Dibutyltin dilaurate | |
Source | CAS Common Chemistry | |
URL | https://commonchemistry.cas.org/detail?cas_rn=77-58-7 | |
Description | CAS Common Chemistry is an open community resource for accessing chemical information. Nearly 500,000 chemical substances from CAS REGISTRY cover areas of community interest, including common and frequently regulated chemicals, and those relevant to high school and undergraduate chemistry classes. This chemical information, curated by our expert scientists, is provided in alignment with our mission as a division of the American Chemical Society. | |
Explanation | The data from CAS Common Chemistry is provided under a CC-BY-NC 4.0 license, unless otherwise stated. | |
Record name | DIBUTYLTIN DILAURATE | |
Source | FDA Global Substance Registration System (GSRS) | |
URL | https://gsrs.ncats.nih.gov/ginas/app/beta/substances/L4061GMT90 | |
Description | The FDA Global Substance Registration System (GSRS) enables the efficient and accurate exchange of information on what substances are in regulated products. Instead of relying on names, which vary across regulatory domains, countries, and regions, the GSRS knowledge base makes it possible for substances to be defined by standardized, scientific descriptions. | |
Explanation | Unless otherwise noted, the contents of the FDA website (www.fda.gov), both text and graphics, are not copyrighted. They are in the public domain and may be republished, reprinted and otherwise used freely by anyone without the need to obtain permission from FDA. Credit to the U.S. Food and Drug Administration as the source is appreciated but not required. | |
Retrosynthesis Analysis
AI-Powered Synthesis Planning: Our tool employs the Template_relevance Pistachio, Template_relevance Bkms_metabolic, Template_relevance Pistachio_ringbreaker, Template_relevance Reaxys, Template_relevance Reaxys_biocatalysis model, leveraging a vast database of chemical reactions to predict feasible synthetic routes.
One-Step Synthesis Focus: Specifically designed for one-step synthesis, it provides concise and direct routes for your target compounds, streamlining the synthesis process.
Accurate Predictions: Utilizing the extensive PISTACHIO, BKMS_METABOLIC, PISTACHIO_RINGBREAKER, REAXYS, REAXYS_BIOCATALYSIS database, our tool offers high-accuracy predictions, reflecting the latest in chemical research and data.
Strategy Settings
Precursor scoring | Relevance Heuristic |
---|---|
Min. plausibility | 0.01 |
Model | Template_relevance |
Template Set | Pistachio/Bkms_metabolic/Pistachio_ringbreaker/Reaxys/Reaxys_biocatalysis |
Top-N result to add to graph | 6 |
Feasible Synthetic Routes
体外研究产品的免责声明和信息
请注意,BenchChem 上展示的所有文章和产品信息仅供信息参考。 BenchChem 上可购买的产品专为体外研究设计,这些研究在生物体外进行。体外研究,源自拉丁语 "in glass",涉及在受控实验室环境中使用细胞或组织进行的实验。重要的是要注意,这些产品没有被归类为药物或药品,他们没有得到 FDA 的批准,用于预防、治疗或治愈任何医疗状况、疾病或疾病。我们必须强调,将这些产品以任何形式引入人类或动物的身体都是法律严格禁止的。遵守这些指南对确保研究和实验的法律和道德标准的符合性至关重要。