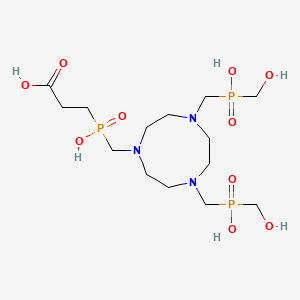
NOPO
描述
作用机制
Target of Action
NOPO, also known as Noopept, primarily targets the brain, specifically the neurotransmission processes . It interacts with acetylcholine signaling pathways and promotes the expression of Brain-Derived Neurotrophic Factor (BDNF) and Nerve Growth Factor (NGF) . In another context, this compound is also known to interact with DNA polymerase η, promoting its ubiquitylation .
Mode of Action
Noopept’s mode of action is multifaceted. It boosts acetylcholine signaling, which is crucial for memory and learning processes . It also promotes the expression of BDNF and NGF, proteins that are essential for brain health, growth, and development . Furthermore, it defends against glutamate toxicity and enhances inhibitory neurotransmission in the brain . In the context of DNA polymerase η, this compound acts as an E3 ubiquitin ligase, promoting the ubiquitylation of the polymerase .
Biochemical Pathways
Noopept affects several biochemical pathways. It enhances acetylcholine signaling, which is a key pathway for memory and learning . It also influences the expression of BDNF and NGF, which are involved in the growth and survival of neurons .
Pharmacokinetics
Noopept has a short half-life (around 5-10 minutes in rats) and is eliminated from the brain within one hour after dosing . Its metabolite, cycloprolylglycine (cPG), is thought to be responsible for Noopept’s longer-term nootropic effects .
Result of Action
The result of Noopept’s action is primarily cognitive enhancement. It has been shown to improve focus, memory, and reduce irritability and emotional instability . In the context of DNA polymerase η, this compound’s action results in enhanced ubiquitylation of the polymerase, which may have implications for DNA repair and genomic stability .
Action Environment
The action of this compound is influenced by various environmental factors. For instance, the efficacy of Noopept may vary depending on the individual’s brain chemistry and overall health . In the context of DNA polymerase η ubiquitylation, the cellular environment and the presence of other proteins may influence this compound’s action .
准备方法
The synthesis of NOPO involves several steps, typically starting with the nitration of propanol. The process can be summarized as follows:
Nitration of Propanol: Propanol is treated with a nitrating agent, such as nitric acid, to introduce a nitro group, forming 3-nitropropanol.
Oxidation: The 3-nitropropanol is then oxidized to form 3-nitrooxypropanol.
Industrial production methods for this compound are designed to be efficient and scalable, ensuring that the compound can be produced in large quantities to meet demand. These methods often involve continuous flow processes and advanced purification techniques to achieve high purity levels .
化学反应分析
NOPO undergoes various chemical reactions, including:
Oxidation: this compound can be further oxidized to form other nitro compounds. Common oxidizing agents include hydrogen peroxide and peracids.
Reduction: this compound can be reduced to form 3-aminopropanol using reducing agents such as lithium aluminum hydride.
Substitution: this compound can undergo nucleophilic substitution reactions, where the nitro group is replaced by other functional groups.
The major products formed from these reactions depend on the specific conditions and reagents used. For example, reduction of this compound typically yields 3-aminopropanol, while substitution reactions can produce a variety of derivatives depending on the nucleophile employed .
科学研究应用
NOPO has a wide range of scientific research applications, including:
相似化合物的比较
NOPO can be compared with other compounds that have similar applications, such as:
属性
IUPAC Name |
3-[[4,7-bis[[hydroxy(hydroxymethyl)phosphoryl]methyl]-1,4,7-triazonan-1-yl]methyl-hydroxyphosphoryl]propanoic acid | |
---|---|---|
Details | Computed by Lexichem TK 2.7.0 (PubChem release 2021.05.07) | |
Source | PubChem | |
URL | https://pubchem.ncbi.nlm.nih.gov | |
Description | Data deposited in or computed by PubChem | |
InChI |
InChI=1S/C14H32N3O10P3/c18-12-29(24,25)10-16-4-2-15(9-28(22,23)8-1-14(20)21)3-5-17(7-6-16)11-30(26,27)13-19/h18-19H,1-13H2,(H,20,21)(H,22,23)(H,24,25)(H,26,27) | |
Details | Computed by InChI 1.0.6 (PubChem release 2021.05.07) | |
Source | PubChem | |
URL | https://pubchem.ncbi.nlm.nih.gov | |
Description | Data deposited in or computed by PubChem | |
InChI Key |
ZNYVGVMHKCUCAT-UHFFFAOYSA-N | |
Details | Computed by InChI 1.0.6 (PubChem release 2021.05.07) | |
Source | PubChem | |
URL | https://pubchem.ncbi.nlm.nih.gov | |
Description | Data deposited in or computed by PubChem | |
Canonical SMILES |
C1CN(CCN(CCN1CP(=O)(CCC(=O)O)O)CP(=O)(CO)O)CP(=O)(CO)O | |
Details | Computed by OEChem 2.3.0 (PubChem release 2021.05.07) | |
Source | PubChem | |
URL | https://pubchem.ncbi.nlm.nih.gov | |
Description | Data deposited in or computed by PubChem | |
Molecular Formula |
C14H32N3O10P3 | |
Details | Computed by PubChem 2.1 (PubChem release 2021.05.07) | |
Source | PubChem | |
URL | https://pubchem.ncbi.nlm.nih.gov | |
Description | Data deposited in or computed by PubChem | |
Molecular Weight |
495.34 g/mol | |
Details | Computed by PubChem 2.1 (PubChem release 2021.05.07) | |
Source | PubChem | |
URL | https://pubchem.ncbi.nlm.nih.gov | |
Description | Data deposited in or computed by PubChem | |
Retrosynthesis Analysis
AI-Powered Synthesis Planning: Our tool employs the Template_relevance Pistachio, Template_relevance Bkms_metabolic, Template_relevance Pistachio_ringbreaker, Template_relevance Reaxys, Template_relevance Reaxys_biocatalysis model, leveraging a vast database of chemical reactions to predict feasible synthetic routes.
One-Step Synthesis Focus: Specifically designed for one-step synthesis, it provides concise and direct routes for your target compounds, streamlining the synthesis process.
Accurate Predictions: Utilizing the extensive PISTACHIO, BKMS_METABOLIC, PISTACHIO_RINGBREAKER, REAXYS, REAXYS_BIOCATALYSIS database, our tool offers high-accuracy predictions, reflecting the latest in chemical research and data.
Strategy Settings
Precursor scoring | Relevance Heuristic |
---|---|
Min. plausibility | 0.01 |
Model | Template_relevance |
Template Set | Pistachio/Bkms_metabolic/Pistachio_ringbreaker/Reaxys/Reaxys_biocatalysis |
Top-N result to add to graph | 6 |
Feasible Synthetic Routes
Q1: What is the primary mechanism of action of NOPO?
A1: this compound acts as a chelating agent, meaning it binds strongly to metal ions. This property is particularly important for its use with radioactive isotopes like Gallium-68 (⁶⁸Ga) in medical imaging (PET scans) [, , , ].
Q2: How does this compound interact with DNA polymerase η (Polη)?
A2: this compound, along with its Drosophila homolog TRIP, functions as an E3 ubiquitin ligase promoting the ubiquitination of Polη. This interaction enhances Polη's activity in translesion synthesis, a DNA damage tolerance process [].
Q3: Can you elaborate on the role of TRIP/NOPO in genomic stability?
A3: TRIP/NOPO forms an E2-E3 ubiquitination complex with BEN-UEV1A (E2 heterodimer). This complex is crucial for maintaining genomic integrity, especially during the early embryonic development in Drosophila [].
Q4: Does this compound interact with other proteins besides Polη?
A4: Yes, this compound has been found to interact with Bendless (BEN), an E2 ubiquitin-conjugating enzyme in Drosophila. This interaction is essential for proper embryonic development [].
Q5: Is there structural information available for this compound?
A5: While a full spectroscopic characterization is not publicly available, its molecular formula (C12H26N3O9P3) and structure are well-established [, , ].
Q6: What is the stability of this compound under various conditions?
A6: this compound demonstrates remarkable stability across a wide pH range, essential for radiolabeling procedures and in vivo applications [, ].
Q7: Are there specific formulation strategies employed with this compound?
A7: this compound is often conjugated to peptides to target specific tissues or receptors. This conjugation process usually involves amide bond formation at its carboxylic acid moiety [, ].
Q8: Does this compound exhibit any unique material compatibility?
A8: The presence of phosphinic acid groups in this compound's structure contributes to its high hydrophilicity, impacting its biodistribution and pharmacokinetic properties [].
Q9: Does this compound exhibit any catalytic activity?
A9: While this compound's primary function is chelation, its interaction with Polη indirectly influences the catalytic activity of the polymerase in DNA repair [].
Q10: What are the main applications of this compound?
A10: this compound is primarily used in the development of radiopharmaceuticals for PET imaging due to its ability to chelate ⁶⁸Ga efficiently [, , , ].
Q11: Have there been computational studies conducted on this compound?
A11: While specific computational studies are not detailed in the provided abstracts, its structural similarity to other TRAP chelators suggests its potential for computational modeling and QSAR studies [].
Q12: How does the structure of this compound contribute to its selectivity for Gallium-68?
A12: The arrangement of phosphinic acid groups and the triazacyclononane ring in this compound creates a coordination environment highly favorable for Ga3+ binding, surpassing carboxylate-based chelators like NOTA and DOTA [, ].
Q13: What is known about the pharmacokinetic profile of this compound conjugates?
A13: this compound conjugates tend to be more hydrophilic than DOTA or NODAGA counterparts, leading to rapid renal clearance, a desirable trait for minimizing radiation exposure [].
Q14: Has this compound been used in preclinical or clinical settings?
A14: Preclinical studies using ⁶⁸Ga-labeled this compound-peptide conjugates have shown promising results in targeting various receptors, including somatostatin receptors in neuroendocrine tumors and αvβ3 integrin in melanoma [, , ].
Q15: Are there specific drug delivery strategies being explored with this compound?
A15: The conjugation of this compound to various targeting peptides exemplifies its use in targeted drug delivery approaches, enhancing the accumulation of the radiotracer in desired tissues [, ].
Q16: What is the impact of metal ion contaminants on this compound labeling?
A16: While carboxylate-based chelators are susceptible to interference from metal ions like Zn2+, this compound exhibits superior resistance and can even displace Zn2+ from its coordination sphere, ensuring efficient ⁶⁸Ga labeling even in the presence of contaminants [].
体外研究产品的免责声明和信息
请注意,BenchChem 上展示的所有文章和产品信息仅供信息参考。 BenchChem 上可购买的产品专为体外研究设计,这些研究在生物体外进行。体外研究,源自拉丁语 "in glass",涉及在受控实验室环境中使用细胞或组织进行的实验。重要的是要注意,这些产品没有被归类为药物或药品,他们没有得到 FDA 的批准,用于预防、治疗或治愈任何医疗状况、疾病或疾病。我们必须强调,将这些产品以任何形式引入人类或动物的身体都是法律严格禁止的。遵守这些指南对确保研究和实验的法律和道德标准的符合性至关重要。