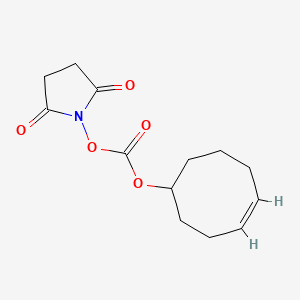
TCO-NHS 酯
描述
TCO-NHS ester is an amine-reactive building block used to modify primary amine-containing molecules . It is used for the modification of amine-containing molecules in organic media . It is supplied as a single axial (minor, rel - (1S-2S-5E-pS)) diastereomer .
Synthesis Analysis
A simple and convenient method for the synthesis of N-hydroxysuccinimide ester is developed using triphosgene as an acid activator . Several aromatic and aliphatic N-hydroxysuccinimide esters are prepared from their corresponding carboxylic acids at room temperature in good yields in a rapid process using triphosgene .Molecular Structure Analysis
The molecular formula of TCO-NHS ester is C13H17NO5 . The InChI code is 1S/C13H17NO5/c15-11-8-9-12 (16)14 (11)19-13 (17)18-10-6-4-2-1-3-5-7-10/h1-2,10H,3-9H2 . The molecular weight is 267.28 g/mol .Chemical Reactions Analysis
TCO-NHS ester reacts specifically and efficiently with a primary amine (e.g., side chain of lysine residues or aminosilane-coated surfaces) at pH 7-9 to form a covalent bond . This reaction is based on the rapid and bio-orthogonal inverse electron demand Diels–Alder reaction between tetrazine (Tz) and trans-cyclooctene (TCO) .Physical And Chemical Properties Analysis
TCO-NHS ester has a molecular weight of 267.28 g/mol . It is a solid substance with a density of 1.27g/cm3 .科学研究应用
Bioconjugation and Protein Labeling
TCO-NHS ester is an axial trans-cyclooctene (a-TCO) used for bioconjugation and protein labeling . It has an amine-reactive NHS ester, and the a-TCO reacts rapidly with tetrazines through Diels–Alder reactions, a type of bioorthogonal, copper-free click chemistry .
Protein-Protein Conjugation
TCO-NHS ester is used in protein-protein conjugation . This application allows for the formation of covalent bonds between proteins, enabling the study of protein interactions and functions.
Protein-Antibody Conjugation
Another application of TCO-NHS ester is in protein-antibody conjugation . This process is crucial in the development of antibody-based therapeutics and diagnostics.
Protein-Small Molecule Conjugation
TCO-NHS ester is also used in protein-small molecule conjugation . This technique is often used in drug delivery systems where a therapeutic small molecule is attached to a protein carrier.
Radiolabeling
TCO-NHS ester is used in 18F radiolabeling . This is a critical process in the production of radiopharmaceuticals for positron emission tomography (PET) imaging.
Protein-Oligonucleotide Conjugation
TCO-NHS ester is used in protein-oligonucleotide conjugation . This technique is used in the development of DNA and RNA aptamers, which have applications in therapeutics and diagnostics.
Surface Modification
Lastly, TCO-NHS ester is used in surface modification . This process is used to alter the surface properties of materials for various applications, including biosensors and biochips.
未来方向
TCO-NHS ester has shown promise in the field of bioconjugation and protein labeling . Its use in the synthesis of PROTACs suggests potential applications in targeted therapy . Furthermore, its role in the inverse electron demand Diels–Alder reaction for pretargeted PET imaging and radioimmunotherapy indicates potential future directions in oncology .
属性
IUPAC Name |
[(4E)-cyclooct-4-en-1-yl] (2,5-dioxopyrrolidin-1-yl) carbonate | |
---|---|---|
Source | PubChem | |
URL | https://pubchem.ncbi.nlm.nih.gov | |
Description | Data deposited in or computed by PubChem | |
InChI |
InChI=1S/C13H17NO5/c15-11-8-9-12(16)14(11)19-13(17)18-10-6-4-2-1-3-5-7-10/h1-2,10H,3-9H2/b2-1+ | |
Source | PubChem | |
URL | https://pubchem.ncbi.nlm.nih.gov | |
Description | Data deposited in or computed by PubChem | |
InChI Key |
OUGQJOKGFAIFAQ-OWOJBTEDSA-N | |
Source | PubChem | |
URL | https://pubchem.ncbi.nlm.nih.gov | |
Description | Data deposited in or computed by PubChem | |
Canonical SMILES |
C1CC=CCCC(C1)OC(=O)ON2C(=O)CCC2=O | |
Source | PubChem | |
URL | https://pubchem.ncbi.nlm.nih.gov | |
Description | Data deposited in or computed by PubChem | |
Isomeric SMILES |
C1C/C=C/CCC(C1)OC(=O)ON2C(=O)CCC2=O | |
Source | PubChem | |
URL | https://pubchem.ncbi.nlm.nih.gov | |
Description | Data deposited in or computed by PubChem | |
Molecular Formula |
C13H17NO5 | |
Source | PubChem | |
URL | https://pubchem.ncbi.nlm.nih.gov | |
Description | Data deposited in or computed by PubChem | |
Molecular Weight |
267.28 g/mol | |
Source | PubChem | |
URL | https://pubchem.ncbi.nlm.nih.gov | |
Description | Data deposited in or computed by PubChem | |
Product Name |
TCO-NHS ester | |
CAS RN |
1191901-33-3 | |
Record name | CYCLOOCT-4-ENYL 2,5-DIOXOPYRROLIDIN-1-YL CARBONATE, (E)- | |
Source | FDA Global Substance Registration System (GSRS) | |
URL | https://gsrs.ncats.nih.gov/ginas/app/beta/substances/2BX65M7XV6 | |
Description | The FDA Global Substance Registration System (GSRS) enables the efficient and accurate exchange of information on what substances are in regulated products. Instead of relying on names, which vary across regulatory domains, countries, and regions, the GSRS knowledge base makes it possible for substances to be defined by standardized, scientific descriptions. | |
Explanation | Unless otherwise noted, the contents of the FDA website (www.fda.gov), both text and graphics, are not copyrighted. They are in the public domain and may be republished, reprinted and otherwise used freely by anyone without the need to obtain permission from FDA. Credit to the U.S. Food and Drug Administration as the source is appreciated but not required. | |
Retrosynthesis Analysis
AI-Powered Synthesis Planning: Our tool employs the Template_relevance Pistachio, Template_relevance Bkms_metabolic, Template_relevance Pistachio_ringbreaker, Template_relevance Reaxys, Template_relevance Reaxys_biocatalysis model, leveraging a vast database of chemical reactions to predict feasible synthetic routes.
One-Step Synthesis Focus: Specifically designed for one-step synthesis, it provides concise and direct routes for your target compounds, streamlining the synthesis process.
Accurate Predictions: Utilizing the extensive PISTACHIO, BKMS_METABOLIC, PISTACHIO_RINGBREAKER, REAXYS, REAXYS_BIOCATALYSIS database, our tool offers high-accuracy predictions, reflecting the latest in chemical research and data.
Strategy Settings
Precursor scoring | Relevance Heuristic |
---|---|
Min. plausibility | 0.01 |
Model | Template_relevance |
Template Set | Pistachio/Bkms_metabolic/Pistachio_ringbreaker/Reaxys/Reaxys_biocatalysis |
Top-N result to add to graph | 6 |
Feasible Synthetic Routes
Q & A
Q1: How does TCO-NHS ester contribute to the pretargeted imaging strategy described in the research?
A1: TCO-NHS ester plays a crucial role in the bioorthogonal click chemistry employed in this imaging strategy. The researchers first modify an antibody targeting the PD-L1 protein with TCO-NHS ester. This modification allows the antibody to conjugate with a Tetrazine-functionalized imaging agent through a rapid and highly specific Diels-Alder reaction in vivo. [] This two-step approach minimizes off-target effects and enhances the signal-to-noise ratio for improved tumor imaging.
体外研究产品的免责声明和信息
请注意,BenchChem 上展示的所有文章和产品信息仅供信息参考。 BenchChem 上可购买的产品专为体外研究设计,这些研究在生物体外进行。体外研究,源自拉丁语 "in glass",涉及在受控实验室环境中使用细胞或组织进行的实验。重要的是要注意,这些产品没有被归类为药物或药品,他们没有得到 FDA 的批准,用于预防、治疗或治愈任何医疗状况、疾病或疾病。我们必须强调,将这些产品以任何形式引入人类或动物的身体都是法律严格禁止的。遵守这些指南对确保研究和实验的法律和道德标准的符合性至关重要。