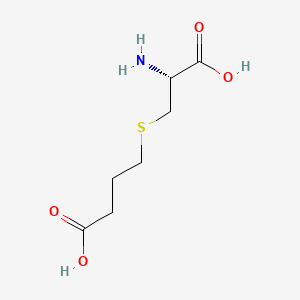
S-(3-Carboxypropyl)-L-cysteine
描述
S-(3-Carboxypropyl)-L-cysteine (CPC) is a modified cysteine derivative with a 3-carboxypropyl thioether group attached to the sulfur atom of L-cysteine. Its molecular formula is C₇H₁₃NO₄S (molecular weight: 207.24 g/mol), and it is characterized by two carboxylic acid groups, making it highly polar and water-soluble . CPC is noted for its specific inhibitory activity against cystathionine γ-lyase (CSE), a key enzyme in hydrogen sulfide (H₂S) biosynthesis. By blocking CSE-dependent H₂S production, CPC has been utilized in studies exploring oxidative stress, cardiovascular diseases, and microbial metabolism .
准备方法
Synthetic Routes and Reaction Conditions: The synthesis of S-(3-Carboxypropyl)-L-cysteine typically involves the reaction of L-cysteine with 3-bromopropionic acid under basic conditions. The reaction proceeds through a nucleophilic substitution mechanism, where the thiol group of cysteine attacks the bromine atom of 3-bromopropionic acid, resulting in the formation of the desired product.
Industrial Production Methods: Industrial production of this compound can be achieved through similar synthetic routes, often optimized for large-scale production. This may involve the use of continuous flow reactors and advanced purification techniques to ensure high yield and purity of the final product.
化学反应分析
Types of Reactions:
Oxidation: S-(3-Carboxypropyl)-L-cysteine can undergo oxidation reactions, where the thiol group is converted to a sulfoxide or sulfone.
Reduction: The compound can also be reduced, typically involving the reduction of the carboxyl group to an alcohol.
Substitution: Nucleophilic substitution reactions can occur at the sulfur atom, leading to the formation of various derivatives.
Common Reagents and Conditions:
Oxidation: Common oxidizing agents include hydrogen peroxide and potassium permanganate.
Reduction: Reducing agents such as lithium aluminum hydride or sodium borohydride are often used.
Substitution: Nucleophiles like alkyl halides or acyl chlorides can be used under basic conditions.
Major Products:
Oxidation: Sulfoxides and sulfones.
Reduction: Alcohol derivatives.
Substitution: Various alkyl or acyl derivatives.
科学研究应用
Inhibition Kinetics
Research indicates that S-(3-Carboxypropyl)-L-cysteine exhibits inhibition constants () of approximately 50 ± 3 µM for the γ-elimination reaction of cystathionine and 180 ± 15 µM for H₂S synthesis from cysteine . This specificity suggests its potential for selective modulation of H₂S synthesis without affecting other related enzymes, such as cystathionine β-synthase and mercaptopyruvate sulfurtransferase.
Potential Therapeutic Uses
The ability of this compound to inhibit CSE has implications for several therapeutic areas:
- Neurological Disorders : Given the role of H₂S in neuroprotection and neurotransmission, this compound may be explored as a treatment option for neurodegenerative diseases where H₂S signaling is disrupted.
- Cancer Treatment : By modulating H₂S levels, this compound could influence tumor microenvironments and cancer cell metabolism, warranting further investigation in oncology settings.
- Cardiovascular Health : H₂S is known to have protective cardiovascular effects; thus, controlling its synthesis through this compound may offer new strategies for managing heart diseases.
Structural Insights
Recent structural studies have provided insights into the interaction between this compound and CSE. The crystal structure reveals that the compound forms a stable aminoacrylate intermediate during the inhibition process, which supports its role as a selective inhibitor . This structural information is crucial for guiding the design of more potent derivatives.
In Vitro Studies
In vitro experiments have demonstrated that this compound can effectively reduce transsulfuration flux by 80–90% in cultured cells, indicating its strong inhibitory effect on CSE activity . This reduction was monitored using radiolabeled methionine transfer to glutathione (GSH), providing a quantitative measure of its impact on cellular metabolism.
Comparative Studies
Comparative studies involving other known inhibitors such as propargylglycine (PPG) have highlighted the unique properties of this compound. Unlike PPG, which shows variable efficacy based on substrate addition order, this compound maintains consistent inhibitory effects regardless of the experimental conditions .
Summary Table of Key Findings
Application Area | Key Findings |
---|---|
Inhibition Kinetics | values: 50 ± 3 µM (cystathionine), 180 ± 15 µM (H₂S synthesis) |
Neurological Disorders | Potential neuroprotective effects through modulation of H₂S signaling |
Cancer Treatment | May influence tumor microenvironments; requires further investigation |
Cardiovascular Health | Possible strategies for managing heart diseases via H₂S control |
Structural Insights | Forms stable aminoacrylate intermediate; supports selective inhibition |
作用机制
The mechanism of action of S-(3-Carboxypropyl)-L-cysteine involves its interaction with cellular thiol groups and enzymes. The carboxypropyl group can modulate the reactivity of the cysteine moiety, affecting its ability to participate in redox reactions and enzyme catalysis. This modulation can influence various cellular pathways, including those involved in oxidative stress response and signal transduction.
相似化合物的比较
Comparison with Structurally Related Compounds
Carboxy-Alkyl Cysteine Derivatives
These compounds feature a carboxylic acid group on the alkyl chain attached to cysteine. Structural variations in chain length and carboxyl group position significantly influence their biological roles.
Key Observations :
- Chain Length and Carboxyl Position : CPC’s 3-carboxypropyl group provides optimal steric and electronic interactions with CSE, unlike shorter-chain derivatives (e.g., S-(2-Carboxyethyl)-L-cysteine) .
- Synthesis : 1-CEC and 1-CPC are synthesized via alkylation of cysteine with brominated carboxylic acids, highlighting the role of carboxyl group placement in reactivity .
Alkyl Cysteine Derivatives (Non-Carboxylated)
These lack carboxyl groups but share the cysteine backbone with variable alkyl chains.
Key Observations :
- Toxicity Mechanisms : DCVC and related halogenated derivatives are metabolized by renal β-lyases to generate toxic thioketenes, leading to mitochondrial dysfunction and cell death . CPC lacks this bioactivation pathway due to its carboxyl group.
- Microbial Interactions : S-Propyl-L-cysteine is metabolized by oral microbiota to flavor compounds, illustrating the role of alkyl chain hydrophobicity in microbial substrate specificity .
Mercapturic Acids (N-Acetylated Derivatives)
These are detoxification metabolites of cysteine S-conjugates, featuring N-acetylation.
Key Observations :
- Detoxification Pathway : Mercapturic acids like N-acetyl-S-(2-carboxypropyl)-L-cysteine are excreted in urine, demonstrating the role of acetylation in reducing cysteine conjugate reactivity .
- Biomarker Utility: N-Acetyl-S-(3-amino-3-oxopropyl)cysteine serves as a biomarker for acrylamide exposure, linking structural features to toxicant monitoring .
Functional and Mechanistic Comparisons
Enzymatic Selectivity
- CPC vs. This distinction arises from CPC’s carboxyl group, which prevents β-elimination reactions .
- Substrate Specificity : Renal β-lyases preferentially metabolize halogenated or aromatic cysteine conjugates (e.g., DCVC) over carboxylated derivatives like CPC .
生物活性
S-(3-Carboxypropyl)-L-cysteine (CPC) is a sulfur-containing amino acid derivative that has garnered significant attention in biochemical and pharmacological research due to its unique biological activities. This compound is primarily known for its role as an inhibitor of cystathionine γ-lyase (CSE), an enzyme crucial for the synthesis of hydrogen sulfide (H₂S) in mammals. The modulation of H₂S levels has implications for various physiological processes, including vasodilation, neurotransmission, and potential therapeutic applications in conditions such as hypertension and neurodegenerative diseases.
Chemical Structure and Properties
CPC is characterized by the presence of a carboxypropyl group attached to the sulfur atom of L-cysteine, enhancing its reactivity. Its molecular formula is C₈H₁₃NO₃S, and it can be represented structurally as follows:
The primary mechanism by which CPC exerts its biological activity is through the inhibition of CSE, which catalyzes the conversion of cysteine to H₂S. By inhibiting this enzyme, CPC effectively reduces H₂S production, which can influence various signaling pathways in the body.
Kinetic Studies
Kinetic studies have demonstrated that CPC inhibits the γ-elimination reaction of cystathionine and H₂S synthesis from cysteine with inhibition constants () of 50 ± 3 µM and 180 ± 15 µM, respectively . This specificity highlights CPC's potential as a selective inhibitor compared to other compounds that may not target CSE as effectively.
Inhibition Studies
Research conducted by Yadav et al. (2019) established that CPC specifically inhibits CSE-dependent H₂S synthesis without affecting other enzymes involved in cysteine metabolism . This selectivity suggests that CPC could be utilized in therapeutic strategies aimed at modulating H₂S levels in pathological conditions.
Case Studies
- Hypertension : In animal models, modulation of H₂S levels through CSE inhibition has been linked to alterations in blood pressure regulation. By reducing H₂S synthesis, CPC may contribute to increased vascular resistance, providing a potential avenue for hypertension management.
- Neurodegenerative Diseases : Given the role of H₂S as a neuromodulator, studies indicate that CPC's inhibitory effect on CSE could have implications for neuroprotection. Reduced H₂S levels may mitigate excitotoxicity associated with neurodegenerative disorders.
Applications and Future Directions
CPC's unique properties make it a candidate for further exploration in drug development. Its specificity for CSE opens up possibilities for targeted therapies aimed at conditions where H₂S signaling is disrupted.
Potential Applications:
- Pharmacological Agent : As a selective inhibitor of CSE, CPC could be developed into a drug targeting diseases characterized by dysregulated H₂S levels.
- Research Tool : In biochemical research, CPC can serve as a valuable tool for studying the role of H₂S in various physiological processes.
Comparative Analysis
To better understand the uniqueness of this compound compared to other sulfur-containing amino acids and their derivatives, the following table summarizes key characteristics:
Compound | Inhibitory Activity | Target Enzyme | Therapeutic Potential |
---|---|---|---|
This compound | Yes | Cystathionine γ-lyase | Hypertension, Neurodegeneration |
Propargylglycine | Yes | Cystathionine γ-lyase | General H₂S modulation |
S-Carboxymethyl-L-cysteine | Yes | Various | Mucolytic effects in respiratory diseases |
常见问题
Basic Research Questions
Q. What are the optimal storage conditions for S-(3-Carboxypropyl)-L-cysteine to ensure long-term stability in laboratory settings?
this compound should be stored as a solid powder at -20°C for up to 3 years or 4°C for 2 years. For solutions, store at -80°C (6 months) or -20°C (1 month) to minimize degradation. Avoid repeated freeze-thaw cycles, and ensure airtight containers to prevent moisture absorption or oxidation .
Q. What solvents are recommended for dissolving this compound in experimental setups?
The compound is typically soluble in DMSO (dimethyl sulfoxide). If solubility issues arise, alternative solvents include water, ethanol, or DMF (dimethylformamide). For in vivo studies, prepare stock solutions in DMSO and dilute with PBS or saline to avoid precipitation. Ensure final DMSO concentrations ≤0.1% for biocompatibility .
Q. What synthetic routes are available for this compound, and how do they compare in yield and purity?
The compound is synthesized via nucleophilic substitution using L-cysteine and 3-bromobutyric acid under mild alkaline conditions. Alternative methods employ coupling agents like DCC (dicyclohexylcarbodiimide) and DMAP (dimethylaminopyridine) to enhance yield. Purification via column chromatography (e.g., C18 reverse-phase) or recrystallization achieves >95% purity. Comparative studies show DCC-based methods yield ~80% purity, while bromoacid routes require post-synthetic carboxylation steps .
Advanced Research Questions
Q. How can researchers address contradictions in reported solubility data for this compound across different solvent systems?
Discrepancies arise from pH-dependent ionization of the carboxyl and thiol groups. For example, solubility in water increases at pH >7 due to deprotonation. Validate solubility using HPLC-UV or LC-MS to quantify dissolved fractions. Adjust solvent polarity or use co-solvents (e.g., acetonitrile-water gradients) for recalcitrant samples .
Q. What analytical methods are most effective for quantifying this compound in biological matrices (e.g., tissue homogenates)?
Liquid chromatography with ODS columns (e.g., Zorbax) and UV detection at 375 nm provides sensitivity down to 0.1 µg/mL. For complex matrices, use step-gradient elution (e.g., 0.01 M acetate buffer pH 3.65 with 4–6% acetonitrile). Post-column derivatization with Ellman’s reagent enhances thiol-group detection .
Q. How do structural modifications (e.g., carboxyl chain length) alter the biochemical activity of this compound?
Comparative studies with analogs like S-(1-carboxyethyl)-L-cysteine show that elongation of the carboxyl chain (e.g., 3-carboxypropyl vs. 2-carboxyethyl) enhances metal chelation capacity but reduces membrane permeability. Use molecular docking or isothermal titration calorimetry to quantify interactions with target enzymes (e.g., glutathione synthetase) .
Q. What strategies mitigate oxidative degradation of this compound during long-term experiments?
Degradation is minimized by:
- Storing under argon or nitrogen to prevent thiol oxidation.
- Adding antioxidants (e.g., 1 mM EDTA or 0.1% ascorbic acid).
- Avoiding exposure to UV light or high temperatures (>25°C). Monitor stability via HPLC-MS to detect disulfide byproducts .
Q. Methodological Guidance
- Synthesis Optimization : Use Schlenk-line techniques to exclude moisture and oxygen during coupling reactions. Monitor intermediates via TLC (Rf ~0.3 in ethyl acetate/methanol 4:1) .
- In Vivo Dosing : For animal studies, administer via intraperitoneal injection (10 mg/kg in PBS) or oral gavage (20 mg/kg in 0.5% methylcellulose). Validate bioavailability using plasma LC-MS/MS .
属性
IUPAC Name |
4-[(2R)-2-amino-2-carboxyethyl]sulfanylbutanoic acid | |
---|---|---|
Source | PubChem | |
URL | https://pubchem.ncbi.nlm.nih.gov | |
Description | Data deposited in or computed by PubChem | |
InChI |
InChI=1S/C7H13NO4S/c8-5(7(11)12)4-13-3-1-2-6(9)10/h5H,1-4,8H2,(H,9,10)(H,11,12)/t5-/m0/s1 | |
Source | PubChem | |
URL | https://pubchem.ncbi.nlm.nih.gov | |
Description | Data deposited in or computed by PubChem | |
InChI Key |
WNFNRNDFHINZLV-YFKPBYRVSA-N | |
Source | PubChem | |
URL | https://pubchem.ncbi.nlm.nih.gov | |
Description | Data deposited in or computed by PubChem | |
Canonical SMILES |
C(CC(=O)O)CSCC(C(=O)O)N | |
Source | PubChem | |
URL | https://pubchem.ncbi.nlm.nih.gov | |
Description | Data deposited in or computed by PubChem | |
Isomeric SMILES |
C(CC(=O)O)CSC[C@@H](C(=O)O)N | |
Source | PubChem | |
URL | https://pubchem.ncbi.nlm.nih.gov | |
Description | Data deposited in or computed by PubChem | |
Molecular Formula |
C7H13NO4S | |
Source | PubChem | |
URL | https://pubchem.ncbi.nlm.nih.gov | |
Description | Data deposited in or computed by PubChem | |
Molecular Weight |
207.25 g/mol | |
Source | PubChem | |
URL | https://pubchem.ncbi.nlm.nih.gov | |
Description | Data deposited in or computed by PubChem | |
体外研究产品的免责声明和信息
请注意,BenchChem 上展示的所有文章和产品信息仅供信息参考。 BenchChem 上可购买的产品专为体外研究设计,这些研究在生物体外进行。体外研究,源自拉丁语 "in glass",涉及在受控实验室环境中使用细胞或组织进行的实验。重要的是要注意,这些产品没有被归类为药物或药品,他们没有得到 FDA 的批准,用于预防、治疗或治愈任何医疗状况、疾病或疾病。我们必须强调,将这些产品以任何形式引入人类或动物的身体都是法律严格禁止的。遵守这些指南对确保研究和实验的法律和道德标准的符合性至关重要。