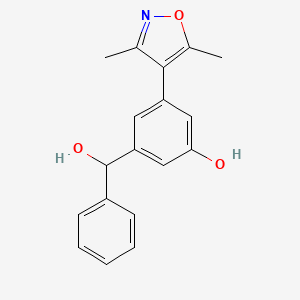
Oxf BD 02
- 点击 快速询问 获取最新报价。
- 提供有竞争力价格的高质量产品,您可以更专注于研究。
描述
OXF BD 02 is a selective inhibitor of the first bromodomain of BRD4 (BRD4 (1)). It is known for its anticancer and anti-inflammatory properties. The compound has shown significant potential in reducing the viability of lung adenocarcinoma cell lines and attenuating the proliferation of MV-4-11 leukemia cells .
科学研究应用
OXF BD 02 has a wide range of scientific research applications:
Chemistry: It is used as a tool compound to study the function of bromodomains and their role in gene regulation.
Biology: The compound is used to investigate the biological pathways involving BRD4 and its impact on cell proliferation and viability.
Medicine: this compound is being explored for its potential therapeutic applications in treating cancers, particularly lung adenocarcinoma and leukemia.
Industry: The compound is used in the development of new drugs and therapeutic agents targeting bromodomains
作用机制
OXF BD 02 exerts its effects by selectively inhibiting the first bromodomain of BRD4. This inhibition disrupts the interaction between BRD4 and acetylated lysine residues on histones, leading to changes in gene expression. The compound exhibits 2-3-fold selectivity for BRD4 (1) over the CBP bromodomain and has little affinity for a range of other bromodomains . This selective inhibition results in reduced cell viability and proliferation in cancer cell lines.
生化分析
Biochemical Properties
Oxf BD 02 plays a significant role in biochemical reactions. It interacts with the first bromodomain of BRD4 . The nature of these interactions is inhibitory, as this compound acts as a selective inhibitor of BRD4(1) .
Cellular Effects
This compound has been found to reduce the viability of lung adenocarcinoma cell lines and attenuate the proliferation of MV-4-11 leukemia cells . It influences cell function by inhibiting BRD4(1), which may impact cell signaling pathways, gene expression, and cellular metabolism .
Molecular Mechanism
The molecular mechanism of this compound involves its binding to the first bromodomain of BRD4, inhibiting its function . This inhibition can lead to changes in gene expression and potentially affect various cellular processes .
准备方法
The synthesis of OXF BD 02 involves the preparation of 3-(3,5-dimethyl-4-isoxazolyl)-5-hydroxy-α-phenylbenzenemethanol. The compound is typically synthesized through a series of chemical reactions that include the formation of the isoxazole ring and subsequent functionalization to introduce the hydroxyl and phenyl groups . Industrial production methods often involve optimizing these reactions to achieve high yields and purity, typically greater than 98% .
化学反应分析
OXF BD 02 undergoes various chemical reactions, including:
Oxidation: The compound can be oxidized under specific conditions to form corresponding oxidized products.
Reduction: Reduction reactions can be performed to modify the functional groups present in the compound.
Substitution: The hydroxyl and phenyl groups in this compound can undergo substitution reactions with various reagents to form different derivatives
Common reagents used in these reactions include oxidizing agents like potassium permanganate, reducing agents like sodium borohydride, and various nucleophiles for substitution reactions. The major products formed from these reactions depend on the specific conditions and reagents used.
相似化合物的比较
OXF BD 02 is unique in its high selectivity for BRD4 (1) compared to other bromodomain inhibitors. Similar compounds include:
JQ1: Another bromodomain inhibitor with broader activity across multiple bromodomains.
I-BET762: A selective inhibitor of BET bromodomains, including BRD2, BRD3, and BRD4.
PFI-1: A selective inhibitor of BRD4 with similar properties to this compound but with different selectivity profiles
This compound stands out due to its specific selectivity for BRD4 (1) and its potent anticancer and anti-inflammatory activities.
属性
IUPAC Name |
3-(3,5-dimethyl-1,2-oxazol-4-yl)-5-[hydroxy(phenyl)methyl]phenol |
Source
|
---|---|---|
Source | PubChem | |
URL | https://pubchem.ncbi.nlm.nih.gov | |
Description | Data deposited in or computed by PubChem | |
InChI |
InChI=1S/C18H17NO3/c1-11-17(12(2)22-19-11)14-8-15(10-16(20)9-14)18(21)13-6-4-3-5-7-13/h3-10,18,20-21H,1-2H3 |
Source
|
Source | PubChem | |
URL | https://pubchem.ncbi.nlm.nih.gov | |
Description | Data deposited in or computed by PubChem | |
InChI Key |
FEQUIPXIENTMJN-UHFFFAOYSA-N |
Source
|
Source | PubChem | |
URL | https://pubchem.ncbi.nlm.nih.gov | |
Description | Data deposited in or computed by PubChem | |
Canonical SMILES |
CC1=C(C(=NO1)C)C2=CC(=CC(=C2)O)C(C3=CC=CC=C3)O |
Source
|
Source | PubChem | |
URL | https://pubchem.ncbi.nlm.nih.gov | |
Description | Data deposited in or computed by PubChem | |
Molecular Formula |
C18H17NO3 |
Source
|
Source | PubChem | |
URL | https://pubchem.ncbi.nlm.nih.gov | |
Description | Data deposited in or computed by PubChem | |
Molecular Weight |
295.3 g/mol |
Source
|
Source | PubChem | |
URL | https://pubchem.ncbi.nlm.nih.gov | |
Description | Data deposited in or computed by PubChem | |
体外研究产品的免责声明和信息
请注意,BenchChem 上展示的所有文章和产品信息仅供信息参考。 BenchChem 上可购买的产品专为体外研究设计,这些研究在生物体外进行。体外研究,源自拉丁语 "in glass",涉及在受控实验室环境中使用细胞或组织进行的实验。重要的是要注意,这些产品没有被归类为药物或药品,他们没有得到 FDA 的批准,用于预防、治疗或治愈任何医疗状况、疾病或疾病。我们必须强调,将这些产品以任何形式引入人类或动物的身体都是法律严格禁止的。遵守这些指南对确保研究和实验的法律和道德标准的符合性至关重要。