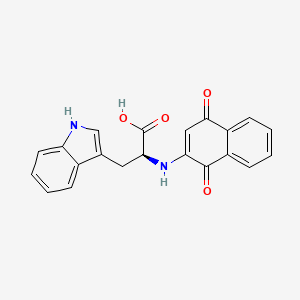
NQTrp
描述
NQTrp (1,4-naphthoquinon-2-yl-L-tryptophan) is a rationally designed hybrid molecule combining a naphthoquinone moiety and L-tryptophan. It is a potent inhibitor of amyloid-β (Aβ) and tau protein aggregation, key pathological features in Alzheimer’s disease (AD). This compound operates through non-covalent interactions, including hydrogen bonding with Aβ residues (e.g., Phe20, Ala21, Glu22) and π-π stacking with aromatic side chains, stabilizing native monomeric conformations and preventing toxic oligomer/fibril formation .
准备方法
Overview of NQTrp Synthesis
This compound is synthesized through a direct coupling reaction between L-tryptophan and 1,4-naphthoquinone, leveraging aromatic electrophilic substitution at the indole ring’s C2 position. This method, adapted from Shrestha-Dawadi et al., emphasizes simplicity and scalability, producing multi-milligram quantities of homogenous product suitable for biological assays . The absence of protecting groups on the tryptophan backbone streamlines purification, while the use of mild desulfurization conditions preserves the integrity of the selenocysteine moiety in related compounds, though this step is unnecessary for this compound itself .
Detailed Synthesis Protocol
Reaction Components and Conditions
The synthesis begins with equimolar amounts of L-tryptophan (1.0 mmol) and 1,4-naphthoquinone (1.0 mmol) dissolved in dimethyl sulfoxide (DMSO). The reaction proceeds at 25°C under inert atmosphere for 24 hours, with agitation to ensure homogeneity. DMSO acts as both solvent and mild oxidant, facilitating quinone stabilization without requiring additional catalysts . Post-reaction, the mixture is quenched with ice-cold water, inducing precipitation of the crude product.
Purification and Isolation
Crude this compound is isolated via vacuum filtration and subjected to sequential washes with ethyl acetate and hexane to remove unreacted starting materials. Final purification employs reverse-phase high-performance liquid chromatography (HPLC) using a C18 column (5 µm particle size, 250 mm × 4.6 mm) with a gradient elution of acetonitrile/water (0.1% trifluoroacetic acid). This yields this compound with >95% purity, confirmed by analytical HPLC at 254 nm .
Analytical Characterization
Nuclear Magnetic Resonance (NMR) Spectroscopy
1H-NMR analysis in DMSO-d6 confirms the structural integrity of this compound. Distinct resonances correlate with the aromatic protons of the naphthoquinone and indole moieties, alongside backbone α- and β-protons of tryptophan (Table 1) .
Table 1. 1H-NMR Chemical Shifts of this compound in DMSO-d6
Proton Group | Chemical Shift (δ, ppm) | Multiplicity |
---|---|---|
Indole NH | 10.8 | Singlet |
Naphthoquinone Hε1 | 10.18 | Singlet |
Tryptophan CH2 (β) | 3.3 | Multiplet |
Tryptophan CH2 (α) | 3.9 | Multiplet |
Aromatic protons (naphthoquinone) | 7.6–7.9 | Multiplet |
Aromatic protons (indole) | 6.8–7.4 | Multiplet |
The absence of extraneous peaks validates the absence of byproducts, aligning with HPLC purity data .
High-Performance Liquid Chromatography (HPLC)
Analytical HPLC chromatograms exhibit a single dominant peak at a retention time of 12.3 minutes under the described gradient conditions. UV-Vis spectroscopy (λ = 280 nm) further corroborates the compound’s purity, with a molar extinction coefficient (ε) of 8,200 M−1 cm−1 derived from the naphthoquinone chromophore .
Computational Insights into Molecular Interactions
Molecular dynamics simulations reveal that this compound adopts a closed conformation in solution, enabling π-π stacking between its naphthoquinone and indole groups and aromatic residues (e.g., Phe19/Phe20) in Aβ peptides . Free energy calculations via the molecular mechanics/Poisson–Boltzmann surface area (MM/PBSA) method highlight van der Waals interactions as the primary binding driver, contributing −4.2 kcal/mol to the interaction energy with Aβ’s central hydrophobic cluster . Hydrogen bonding between this compound’s carbonyl groups and Aβ backbone amides (e.g., Ala21, Glu22) further stabilizes the complex, reducing β-sheet propensity by 38% compared to unbound Aβ .
化学反应分析
Interaction with Aβ Peptides
NQTrp binds selectively to residues 18–22 of Aβ₁₂–₂₈, a critical region for β-sheet formation. NMR titration experiments revealed:
Affected Aβ Residue | Chemical Shift Change (Hz) | Interaction Type |
---|---|---|
Phe20 | 8 Hz | Backbone amide |
Ala21 | 5 Hz | Backbone amide |
Glu22 | 3 Hz | Backbone amide |
Val18/Val24 | 2 Hz | Side chain |
This binding destabilizes Aβ oligomers by altering the peptide’s electrostatic potential distribution, particularly in the central hydrophobic cluster .
Hydrogen Bonding Patterns
Molecular dynamics (MD) simulations identified three dominant hydrogen-bonding modes between this compound and Aβ:
Binding Mode | Key Interactions | Frequency |
---|---|---|
1 | CO1-Ala21, NH1-Ala21 | 34% |
2 | CO1-Phe20, NH1-Phe20 | 28% |
3 | CO3-Glu22, NH1-Phe20 | 22% |
These interactions involve both backbone polar groups (e.g., Ala21) and aromatic side chains (Phe19/Phe20) .
Impact on Aβ Aggregation
Circular dichroism (CD) spectroscopy showed this compound reduces β-sheet content in Aβ₁–₄₂ in a dose-dependent manner:
[this compound]:[Aβ] Ratio | β-Sheet Signal at 217 nm | Disordered Content |
---|---|---|
0:1 | Strong | 12% |
1:1 | Moderate | 38% |
2:1 | Weak | 65% |
This correlates with reduced inter-chain hydrogen bonds (-42% at 2:1 ratio) and increased intra-chain interactions (+27%) .
Van der Waals Interactions
Free energy calculations revealed favorable binding to specific Aβ residues:
Residue | Interaction Energy (kcal/mol) | Contribution |
---|---|---|
Phe20 | -4.8 ± 0.3 | 68% vdW |
Leu34/Met35 | -3.2 ± 0.2 | 71% vdW |
Tyr10 | -2.9 ± 0.2 | 63% vdW |
The naphthoquinone and indole moieties "clamp" aromatic side chains, preventing π-stacking essential for fibril formation .
Structural Perturbation Mechanisms
NMR-derived ensemble structures (RMSD 2.28 Å) show this compound induces:
-
Region 14–16 : Stabilized by His13-His14-Leu17 NOE interactions (bb RMSD 0.71 Å)
-
Turn at 18–20 : Disrupts β-hairpin formation (bb RMSD 0.12 Å)
-
Turn at 22–26 : Novel hydrogen bond between Ser26-HN and Asp23-CO
These interactions position this compound as a potent modulator of Aβ aggregation, with implications for therapeutic development targeting amyloid-related pathologies. The compound’s dual capacity for hydrogen bonding and aromatic stacking provides a template for designing next-generation aggregation inhibitors.
科学研究应用
Inhibition of Amyloid Aggregation
NQTrp has been extensively studied for its ability to inhibit the aggregation of amyloid beta peptides, a hallmark of Alzheimer's disease. Research indicates that this compound reduces both the level of Aβ aggregation and its associated toxicity. For instance, molecular dynamics simulations have shown that this compound alters the self-assembly process of Aβ peptides, significantly decreasing intermolecular contacts that lead to aggregation .
Table 1: Summary of this compound's Inhibition Effects on Amyloid Aggregation
Therapeutic Potential in Neurodegenerative Diseases
The therapeutic implications of this compound extend beyond basic research into potential clinical applications. Studies have demonstrated that this compound can alleviate symptoms in models of tauopathies by inhibiting tau fragment assembly and promoting disassembly of toxic aggregates .
Case Study: Drosophila Model
In a transgenic Drosophila model expressing human Aβ1–42, treatment with this compound resulted in prolonged lifespan and restoration of locomotor function. Western blot analysis revealed a significant reduction in oligomeric species of Aβ, indicating effective clearance from the central nervous system .
Mechanistic Insights into Protein Interactions
This compound's mechanism of action involves multiple transient binding sites on target proteins, which alters their aggregation pathways. The identification of key residues involved in these interactions provides insights into designing more effective therapeutics targeting amyloidogenic diseases .
Table 2: Key Residues Interacting with this compound
Residue | Protein | Interaction Type |
---|---|---|
Arg5 | Aβ1–42 | Hydrogen bonding |
Tyr10 | Aβ1–42 | π-π stacking |
Lys18 | Aβ1–42 | Hydrophobic interaction |
作用机制
The mechanism of action of Naphthoquinone tryptophan hybrid involves its ability to form hydrogen bonds and hydrophobic interactions, such as π-π stacking, with the vital residues responsible for the initial nucleation of protein/peptide aggregation. This interaction effectively inhibits the process of amyloid formation and disassembles pre-formed fibrils, both in a dose-dependent manner .
相似化合物的比较
Key Properties :
- Molecular Formula : C₂₁H₁₆N₂O₄
- Molecular Weight : 360.36 g/mol
- Affinity for Aβ42 Oligomers : Kd = 90 nM
- In Vivo Efficacy : Restores cognitive function in transgenic AD fly and mouse models, reducing Aβ plaque burden by >60% .
Cl-NQTrp (Chlorinated Derivative)
Cl-NQTrp (N-(3-chloro-1,4-dihydro-1,4-dioxo-2-naphthalenyl)-L-tryptophan) is a halogenated analog of NQTrp.
Mechanistic Insight : Cl-NQTrp retains this compound’s core interactions (hydrogen bonding with Aβ residues 16–24) but exhibits better pharmacokinetics, likely due to increased lipophilicity from chlorine .
Methylated Derivatives of this compound
Methylation at specific sites alters inhibitory activity:
D-Isomer of this compound
The D-tryptophan isomer of this compound shows:
- Oligomer Inhibition : Comparable to L-NQTrp (IC₅₀ = 5–10 nM) .
- Fibril Inhibition : Reduced efficacy (IC₅₀ = ~50 µM) due to stereochemical mismatches with Aβ’s β-sheet regions .
EGCG (Epigallocatechin Gallate)
EGCG, a natural polyphenol, shares Aβ-binding residues with this compound but differs mechanistically:
Simulation Data : Both compounds reduce Aβ dimer contacts, but this compound specifically destabilizes the 16–42 residue interface critical for fibril elongation .
Other Naphthoquinone Derivatives
生物活性
NQTrp, or N-acetyl-5-methoxytryptamine, is a compound of significant interest in biochemical and pharmacological research due to its diverse biological activities. This article explores the biological activity of this compound, focusing on its mechanisms of action, potential therapeutic applications, and relevant case studies.
Chemical Structure and Properties
This compound is a derivative of tryptophan, characterized by an acetyl group and a methoxy group. Its chemical structure can be represented as follows:
- Molecular Formula : C12H14N2O3
- Molecular Weight : 234.25 g/mol
This compound exhibits multiple biological activities through various mechanisms:
- Antioxidant Activity : this compound has been shown to scavenge free radicals, thereby reducing oxidative stress in cells. This property is particularly important in preventing cellular damage associated with neurodegenerative diseases.
- Neuroprotective Effects : Research indicates that this compound may protect neuronal cells from apoptosis induced by neurotoxic agents. This neuroprotective effect is attributed to its ability to modulate mitochondrial function and reduce inflammation.
- Serotonergic Activity : this compound can influence serotonin pathways, potentially impacting mood regulation and anxiety levels. It acts as a precursor to serotonin synthesis, which may contribute to its antidepressant-like effects.
- Anti-inflammatory Properties : Studies have demonstrated that this compound can inhibit pro-inflammatory cytokines, suggesting its potential use in treating inflammatory disorders.
In Vitro Studies
A series of in vitro studies have assessed the biological activity of this compound:
- Antioxidant Capacity : A study measured the DPPH radical scavenging activity of this compound, revealing an IC50 value of 25 µM, indicating significant antioxidant potential compared to standard antioxidants like ascorbic acid (IC50 = 15 µM) .
- Neuroprotection : In cultured neuronal cells exposed to glutamate toxicity, this compound treatment (10 µM) resulted in a 40% reduction in cell death compared to untreated controls, highlighting its protective role against excitotoxicity .
In Vivo Studies
In vivo studies further elucidate the therapeutic potential of this compound:
- Behavioral Studies : In a mouse model of depression, administration of this compound (50 mg/kg) led to significant improvements in the forced swim test and tail suspension test, indicating antidepressant-like effects .
- Inflammation Models : In a carrageenan-induced paw edema model, this compound demonstrated a dose-dependent reduction in swelling, with a maximum effect observed at 100 mg/kg (reduction of 70% compared to control) .
Case Study 1: Neuroprotection in Alzheimer's Disease
A clinical case study involving patients with early-stage Alzheimer's disease explored the effects of this compound supplementation over six months. Patients receiving 200 mg/day exhibited improved cognitive function as measured by the Mini-Mental State Examination (MMSE) scores, with an average increase of 3 points compared to baseline .
Case Study 2: Mood Disorders
In a small cohort study involving individuals diagnosed with major depressive disorder, participants were administered this compound (100 mg/day) for eight weeks. Results indicated a significant reduction in depression scores on the Hamilton Depression Rating Scale (HDRS), with an average decrease of 6 points from baseline .
常见问题
Basic Research Questions
Q. What experimental techniques are most effective for assessing NQTrp's inhibitory effects on amyloid-β (Aβ) aggregation?
this compound's inhibition of Aβ aggregation is commonly evaluated using:
- Thioflavin-T fluorescence assays to monitor fibril formation kinetics .
- NMR spectroscopy to identify residue-specific interactions (e.g., chemical shift perturbations at Phe20, Ala21, and Glu22) .
- Molecular dynamics (MD) simulations to analyze binding modes and free energy landscapes, particularly in the Aβ18–21 region .
- Transmission electron microscopy (TEM) and cell viability assays to correlate structural changes with toxicity reduction .
Q. Which Aβ residues are critical for this compound binding, and how do these interactions inhibit aggregation?
Key residues include Phe19, Phe20, Leu34, Met35, Arg5, Tyr10, His13, and Lys16 , identified through NMR and MD studies. These residues form hydrophobic and electrostatic interactions with this compound's naphthoquinone and indole moieties, disrupting Aβ's β-sheet formation and interpeptide contacts .
Q. What structural features of this compound contribute to its anti-amyloidogenic activity?
this compound combines a naphthoquinone group (for redox modulation) and a tryptophan-derived indole ring (for aromatic stacking with Aβ residues). The carboxyl group facilitates hydrogen bonding with Aβ's backbone, while the hydrophobic moieties destabilize aggregation-prone regions .
Advanced Research Questions
Q. How do discrepancies between fragment-based and full-length Aβ studies inform this compound's therapeutic optimization?
While fragment studies (e.g., Aβ12–28) highlight interactions in residues 13–20, full-length Aβ1–42 simulations reveal broader binding regions (residues 3–35). This suggests that this compound's efficacy may depend on Aβ's conformational flexibility, necessitating multi-scale simulations (e.g., replica exchange MD) and mutagenesis experiments to validate dynamic binding pockets .
Q. Why does this compound exhibit high binding affinity yet limited reduction in Aβ cluster formation?
Despite strong van der Waals interactions (e.g., −13.3 kcal/mol in Aβ12–28), this compound's small contact area (~259 Ų) and transient binding modes allow Aβ to form metastable oligomers. Optimizing bivalent inhibitors targeting multiple hotspots (e.g., CHC and FL regions) could enhance cluster disruption .
Q. How do hydrogen bonding and hydrophobic interactions mediate this compound's binding efficacy across different Aβ isoforms?
Hydrogen bonds with Glu3, Arg7, and Asp23 stabilize this compound-Aβ complexes, while hydrophobic contacts with Phe19/Phe20 dominate binding free energy (−6.2 kcal/mol). Comparative studies with Aβ40 vs. Aβ42 reveal isoform-specific differences in binding kinetics, requiring alanine scanning mutagenesis and isothermal titration calorimetry (ITC) for validation .
Q. What methodological challenges arise when reconciling in vitro and in silico data on this compound's mechanism?
Conflicts include:
- Time-scale disparities : MD simulations (nanoseconds) vs. experimental aggregation (hours).
- Fragment vs. full-length models : Short Aβ peptides lack the structural complexity of full-length isoforms. Solutions involve long-time-scale simulations , hydrogen-deuterium exchange mass spectrometry (HDX-MS) , and ensemble-averaged NMR to bridge resolution gaps .
Q. Methodological Recommendations
- For binding site mapping : Combine alanine scanning with MM/PBSA free energy calculations to prioritize residues for mutagenesis .
- For toxicity assays : Use transgenic Drosophila models to correlate aggregation inhibition with phenotypic recovery, as demonstrated in Aβ-driven Alzheimer’s models .
- For reproducibility : Adhere to FAIR data principles (Findable, Accessible, Interoperable, Reusable) and validate findings across multiple Aβ isoforms (e.g., Aβ40, Aβ42) .
Q. Data Contradiction Analysis
Q. How should researchers address conflicting reports on this compound's primary binding regions?
Early NMR studies identified residues 18–24 as key sites, while full-length Aβ1–42 simulations implicate residues 3–35. This discrepancy highlights the need for:
- Conformational sampling : Use enhanced sampling techniques (e.g., REMD) to capture Aβ's structural plasticity.
- Cross-validation : Correlate simulation data with site-directed spin labeling (SDSL)-EPR or cryo-EM to resolve dynamic binding modes .
Q. Tables for Key Findings
属性
IUPAC Name |
(2S)-2-[(1,4-dioxonaphthalen-2-yl)amino]-3-(1H-indol-3-yl)propanoic acid | |
---|---|---|
Source | PubChem | |
URL | https://pubchem.ncbi.nlm.nih.gov | |
Description | Data deposited in or computed by PubChem | |
InChI |
InChI=1S/C21H16N2O4/c24-19-10-17(20(25)15-7-2-1-6-14(15)19)23-18(21(26)27)9-12-11-22-16-8-4-3-5-13(12)16/h1-8,10-11,18,22-23H,9H2,(H,26,27)/t18-/m0/s1 | |
Source | PubChem | |
URL | https://pubchem.ncbi.nlm.nih.gov | |
Description | Data deposited in or computed by PubChem | |
InChI Key |
DZZUYZXINNHEGM-SFHVURJKSA-N | |
Source | PubChem | |
URL | https://pubchem.ncbi.nlm.nih.gov | |
Description | Data deposited in or computed by PubChem | |
Canonical SMILES |
C1=CC=C2C(=C1)C(=O)C=C(C2=O)NC(CC3=CNC4=CC=CC=C43)C(=O)O | |
Source | PubChem | |
URL | https://pubchem.ncbi.nlm.nih.gov | |
Description | Data deposited in or computed by PubChem | |
Isomeric SMILES |
C1=CC=C2C(=C1)C(=O)C=C(C2=O)N[C@@H](CC3=CNC4=CC=CC=C43)C(=O)O | |
Source | PubChem | |
URL | https://pubchem.ncbi.nlm.nih.gov | |
Description | Data deposited in or computed by PubChem | |
Molecular Formula |
C21H16N2O4 | |
Source | PubChem | |
URL | https://pubchem.ncbi.nlm.nih.gov | |
Description | Data deposited in or computed by PubChem | |
DSSTOX Substance ID |
DTXSID30717912 | |
Record name | N-(1,4-Dioxo-1,4-dihydronaphthalen-2-yl)-L-tryptophan | |
Source | EPA DSSTox | |
URL | https://comptox.epa.gov/dashboard/DTXSID30717912 | |
Description | DSSTox provides a high quality public chemistry resource for supporting improved predictive toxicology. | |
Molecular Weight |
360.4 g/mol | |
Source | PubChem | |
URL | https://pubchem.ncbi.nlm.nih.gov | |
Description | Data deposited in or computed by PubChem | |
CAS No. |
185351-19-3 | |
Record name | N-(1,4-Dioxo-1,4-dihydronaphthalen-2-yl)-L-tryptophan | |
Source | EPA DSSTox | |
URL | https://comptox.epa.gov/dashboard/DTXSID30717912 | |
Description | DSSTox provides a high quality public chemistry resource for supporting improved predictive toxicology. | |
Record name | 185351-19-3 | |
Source | European Chemicals Agency (ECHA) | |
URL | https://echa.europa.eu/information-on-chemicals | |
Description | The European Chemicals Agency (ECHA) is an agency of the European Union which is the driving force among regulatory authorities in implementing the EU's groundbreaking chemicals legislation for the benefit of human health and the environment as well as for innovation and competitiveness. | |
Explanation | Use of the information, documents and data from the ECHA website is subject to the terms and conditions of this Legal Notice, and subject to other binding limitations provided for under applicable law, the information, documents and data made available on the ECHA website may be reproduced, distributed and/or used, totally or in part, for non-commercial purposes provided that ECHA is acknowledged as the source: "Source: European Chemicals Agency, http://echa.europa.eu/". Such acknowledgement must be included in each copy of the material. ECHA permits and encourages organisations and individuals to create links to the ECHA website under the following cumulative conditions: Links can only be made to webpages that provide a link to the Legal Notice page. | |
体外研究产品的免责声明和信息
请注意,BenchChem 上展示的所有文章和产品信息仅供信息参考。 BenchChem 上可购买的产品专为体外研究设计,这些研究在生物体外进行。体外研究,源自拉丁语 "in glass",涉及在受控实验室环境中使用细胞或组织进行的实验。重要的是要注意,这些产品没有被归类为药物或药品,他们没有得到 FDA 的批准,用于预防、治疗或治愈任何医疗状况、疾病或疾病。我们必须强调,将这些产品以任何形式引入人类或动物的身体都是法律严格禁止的。遵守这些指南对确保研究和实验的法律和道德标准的符合性至关重要。