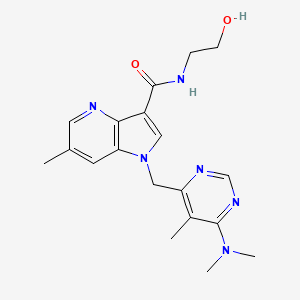
DprE1-IN-2
- 点击 快速询问 获取最新报价。
- 提供有竞争力价格的高质量产品,您可以更专注于研究。
描述
“DprE1-IN-2” is a potent inhibitor of DprE1, an enzyme that plays important roles in the biosynthesis of the mycobacterium cell wall . It has shown great potential in the development of new regimens for tuberculosis (TB) treatment .
Synthesis Analysis
The synthesis of DprE1 inhibitors involves an integrated molecular modeling strategy, which combines computational bioactivity fingerprints and structure-based virtual screening . Two lead compounds, B2 and H3, were identified that could inhibit DprE1 and thus kill Mycobacterium smegmatis in vitro .
Molecular Structure Analysis
The molecular structure of DprE1-IN-2 is complex and unique. It is involved in the synthesis of arabinogalactan, an essential constituent of the Mtb cell wall . The unique structural characteristics for ligand binding and association with DprE2 make developing new clinical compounds challenging .
Chemical Reactions Analysis
DprE1 inhibitors can block arabinan synthesis, thus provoking cell lysis and bacterial death . Both covalent and non-covalent inhibitors have been identified . The chemical reactions involved in the inhibition of DprE1 are complex and involve multiple steps .
Physical And Chemical Properties Analysis
The physical and chemical properties of DprE1-IN-2 are complex. It has a molecular weight of 368.43 and a chemical formula of C19H24N6O2 . It is a solid substance with a solubility of ≥ 33 mg/mL in DMSO .
科学研究应用
Targeting Drug-Resistant Tuberculosis
DprE1-IN-2 is a promising candidate for tackling drug-resistant tuberculosis (TB). As a potent inhibitor of the DprE1 enzyme, it disrupts the biosynthesis of mycobacterial cell walls, which is crucial for the survival of the TB-causing bacterium, Mycobacterium tuberculosis . This makes it a valuable asset in the development of new anti-TB drugs, especially against multidrug-resistant (MDR-TB) and extensively drug-resistant (XDR-TB) strains.
Inhibition of Cell Wall Biosynthesis
The DprE1 enzyme plays a vital role in the synthesis of arabinogalactans and lipoarabinomannans, key components of the mycobacterial cell wall . DprE1-IN-2, by inhibiting this enzyme, halts the production of these essential molecules, thereby weakening the bacterial cell wall and leading to the death of the bacteria.
Computational Drug Design
DprE1-IN-2 has been identified through computational approaches, such as in-silico screening and molecular docking studies . These methods allow for the rapid identification of potential inhibitors based on the enzyme’s structure, speeding up the drug discovery process.
Pharmacophore Model Development
Researchers have utilized e-pharmacophore models to design potential DprE1 inhibitors. DprE1-IN-2 can serve as a reference compound for developing such models, which are crucial for predicting the binding affinity of novel compounds .
Understanding Enzyme-Ligand Interactions
The study of DprE1-IN-2 provides insights into the non-covalent interactions between the enzyme and its inhibitors. This knowledge is essential for the rational design of new drugs with improved efficacy and reduced side effects .
Antitubercular Assays
DprE1-IN-2 has been subjected to in vitro antitubercular assays to determine its efficacy against the TB bacterium. These assays are critical for assessing the therapeutic potential of new drug candidates before advancing to clinical trials .
ADMET Property Analysis
The physicochemical and ADMET (absorption, distribution, metabolism, excretion, and toxicity) properties of DprE1-IN-2 have been computationally analyzed. Understanding these properties is important for optimizing the drug’s pharmacokinetic profile and minimizing toxicity .
Contribution to Multistage Vaccine Development
While not a direct application of DprE1-IN-2, the understanding of DprE1’s role in mycobacterial cell wall biosynthesis contributes to the development of multistage vaccines. These vaccines target different stages of TB infection and could potentially control reactivation from a latent state .
安全和危害
属性
IUPAC Name |
1-[[6-(dimethylamino)-5-methylpyrimidin-4-yl]methyl]-N-(2-hydroxyethyl)-6-methylpyrrolo[3,2-b]pyridine-3-carboxamide |
Source
|
---|---|---|
Source | PubChem | |
URL | https://pubchem.ncbi.nlm.nih.gov | |
Description | Data deposited in or computed by PubChem | |
InChI |
InChI=1S/C19H24N6O2/c1-12-7-16-17(21-8-12)14(19(27)20-5-6-26)9-25(16)10-15-13(2)18(24(3)4)23-11-22-15/h7-9,11,26H,5-6,10H2,1-4H3,(H,20,27) |
Source
|
Source | PubChem | |
URL | https://pubchem.ncbi.nlm.nih.gov | |
Description | Data deposited in or computed by PubChem | |
InChI Key |
BLNAAWXUNQHVNT-UHFFFAOYSA-N |
Source
|
Source | PubChem | |
URL | https://pubchem.ncbi.nlm.nih.gov | |
Description | Data deposited in or computed by PubChem | |
Canonical SMILES |
CC1=CC2=C(C(=CN2CC3=C(C(=NC=N3)N(C)C)C)C(=O)NCCO)N=C1 |
Source
|
Source | PubChem | |
URL | https://pubchem.ncbi.nlm.nih.gov | |
Description | Data deposited in or computed by PubChem | |
Molecular Formula |
C19H24N6O2 |
Source
|
Source | PubChem | |
URL | https://pubchem.ncbi.nlm.nih.gov | |
Description | Data deposited in or computed by PubChem | |
Molecular Weight |
368.4 g/mol |
Source
|
Source | PubChem | |
URL | https://pubchem.ncbi.nlm.nih.gov | |
Description | Data deposited in or computed by PubChem | |
Product Name |
DprE1-IN-2 |
体外研究产品的免责声明和信息
请注意,BenchChem 上展示的所有文章和产品信息仅供信息参考。 BenchChem 上可购买的产品专为体外研究设计,这些研究在生物体外进行。体外研究,源自拉丁语 "in glass",涉及在受控实验室环境中使用细胞或组织进行的实验。重要的是要注意,这些产品没有被归类为药物或药品,他们没有得到 FDA 的批准,用于预防、治疗或治愈任何医疗状况、疾病或疾病。我们必须强调,将这些产品以任何形式引入人类或动物的身体都是法律严格禁止的。遵守这些指南对确保研究和实验的法律和道德标准的符合性至关重要。